The water - Soluble Vitamins ( Francene Steinberg and Robert B. Rucker) | Trường Đại học sư phạm kỹ thuật TP Hồ Chí Minh
The water-soluble vitamins consist of a mixed group of chemical compounds. Their classification into specific chemical groups depends on both chemical characteristics and functions. Tài liệu giúp bạn tham khảo, ôn tập và đạt kết quả cao. Mời bạn đọc đón xem!
Môn: Tiếng Anh - HP1
Trường: Đại học Sư phạm Kỹ thuật Thành phố Hồ Chí Minh
Thông tin:
Tác giả:
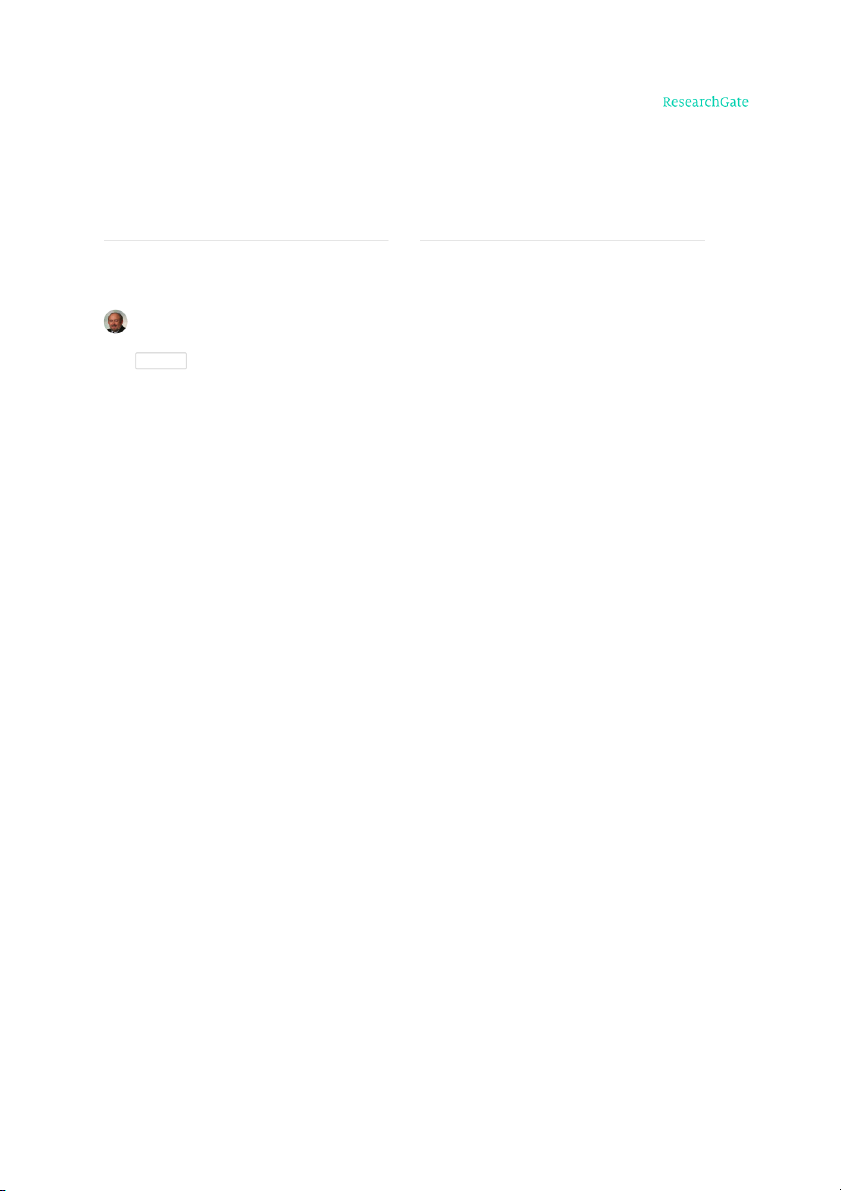
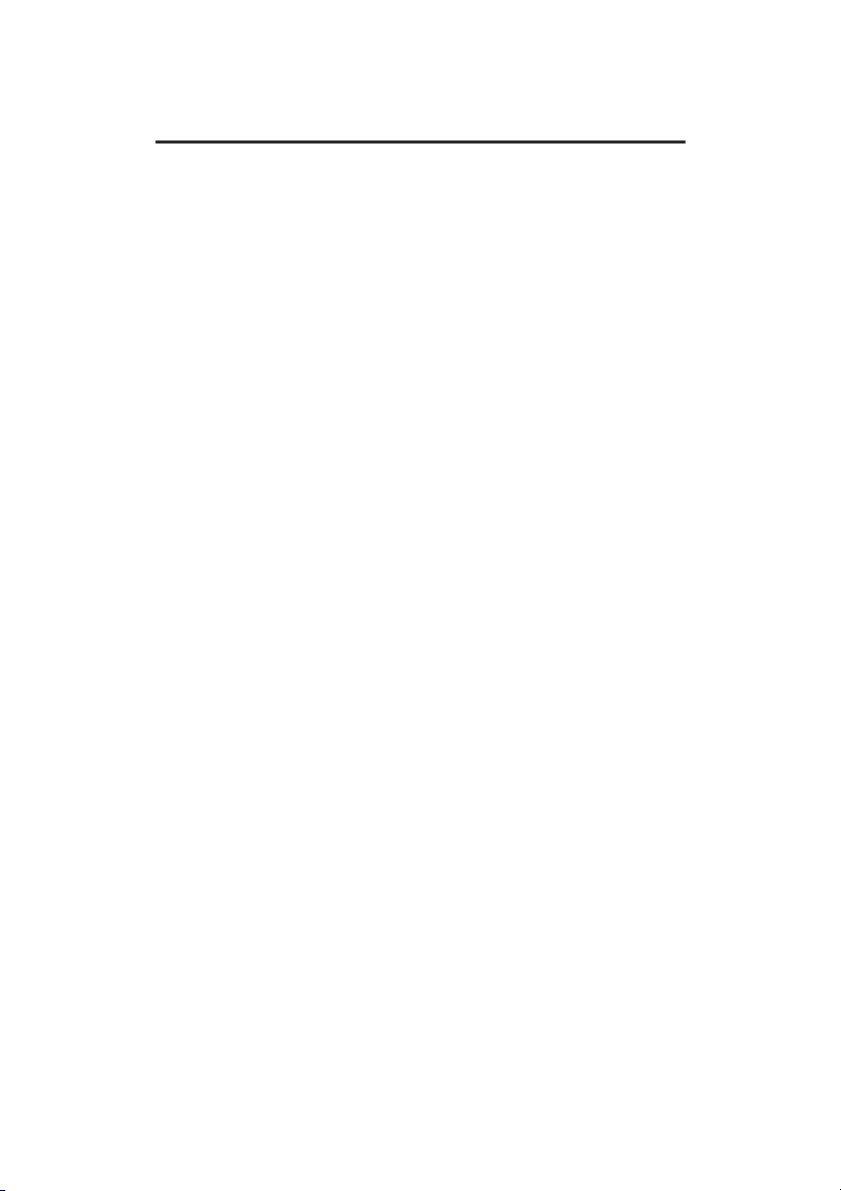
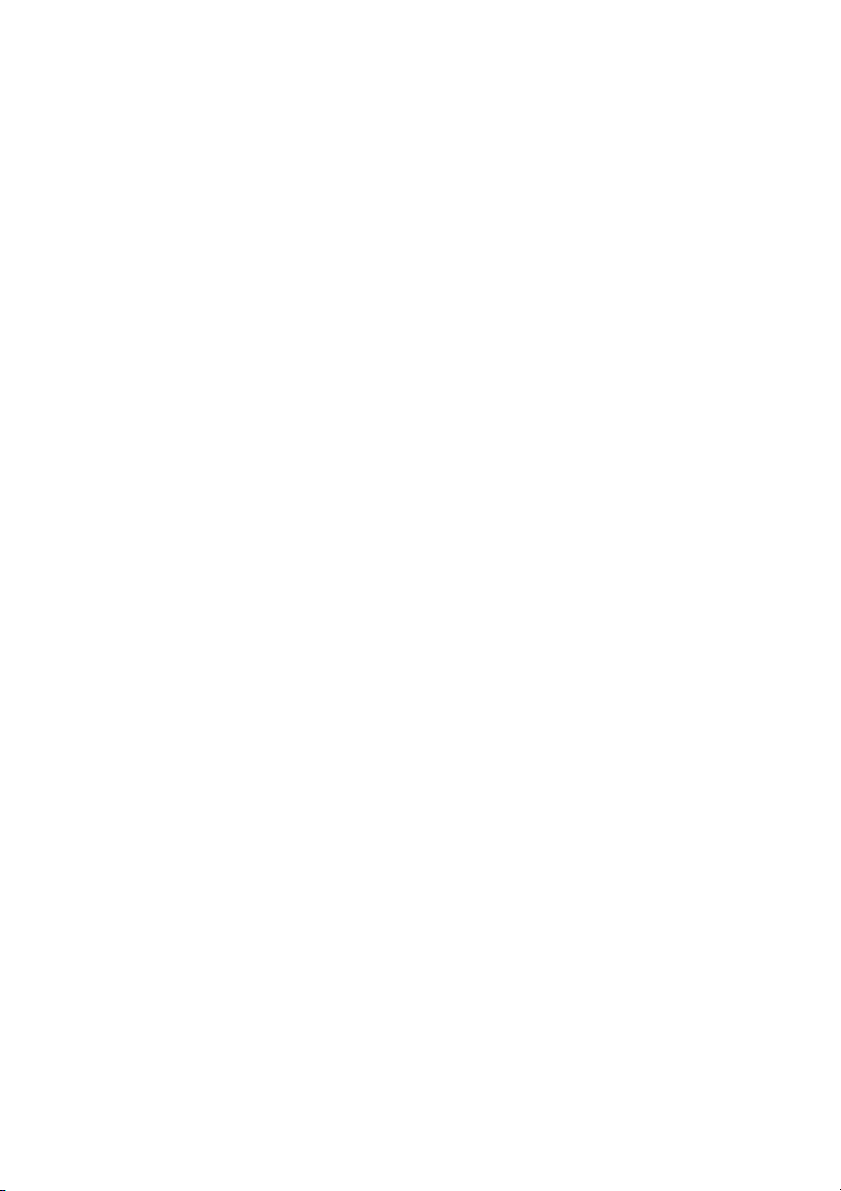
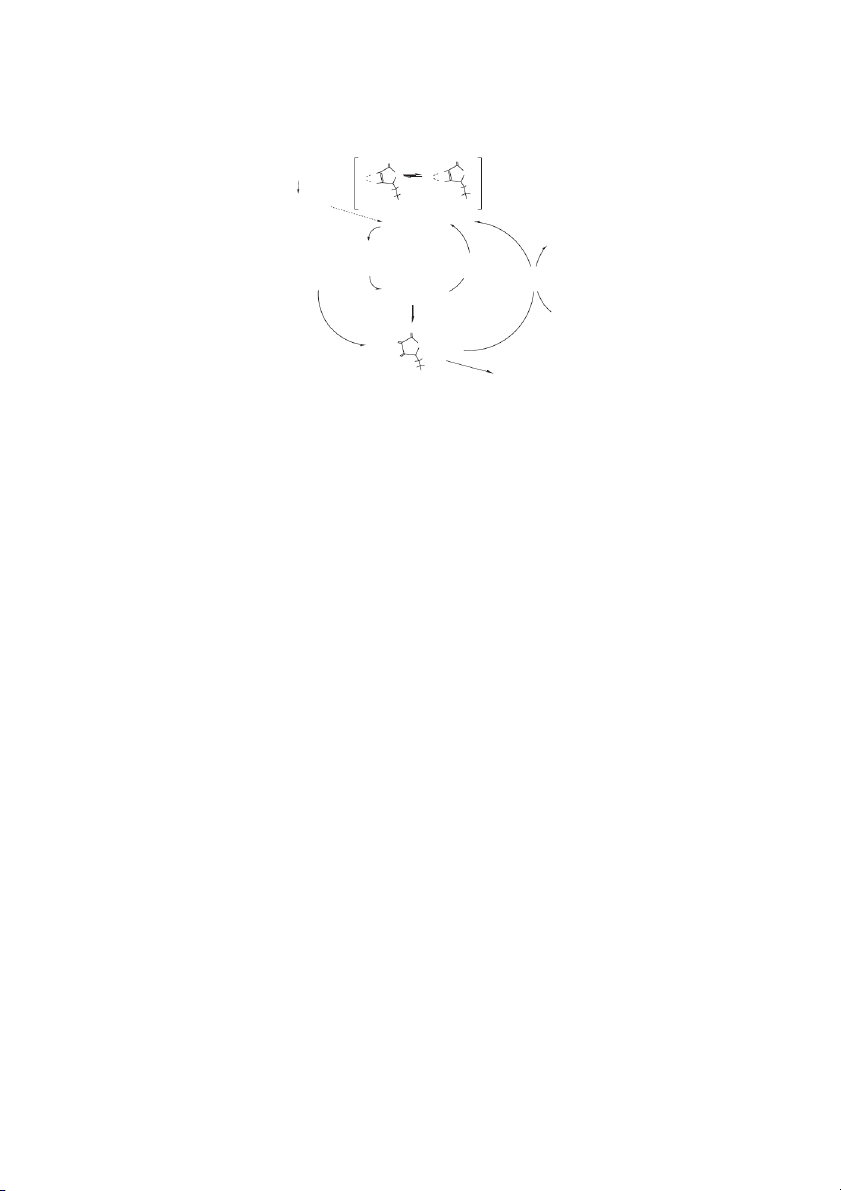
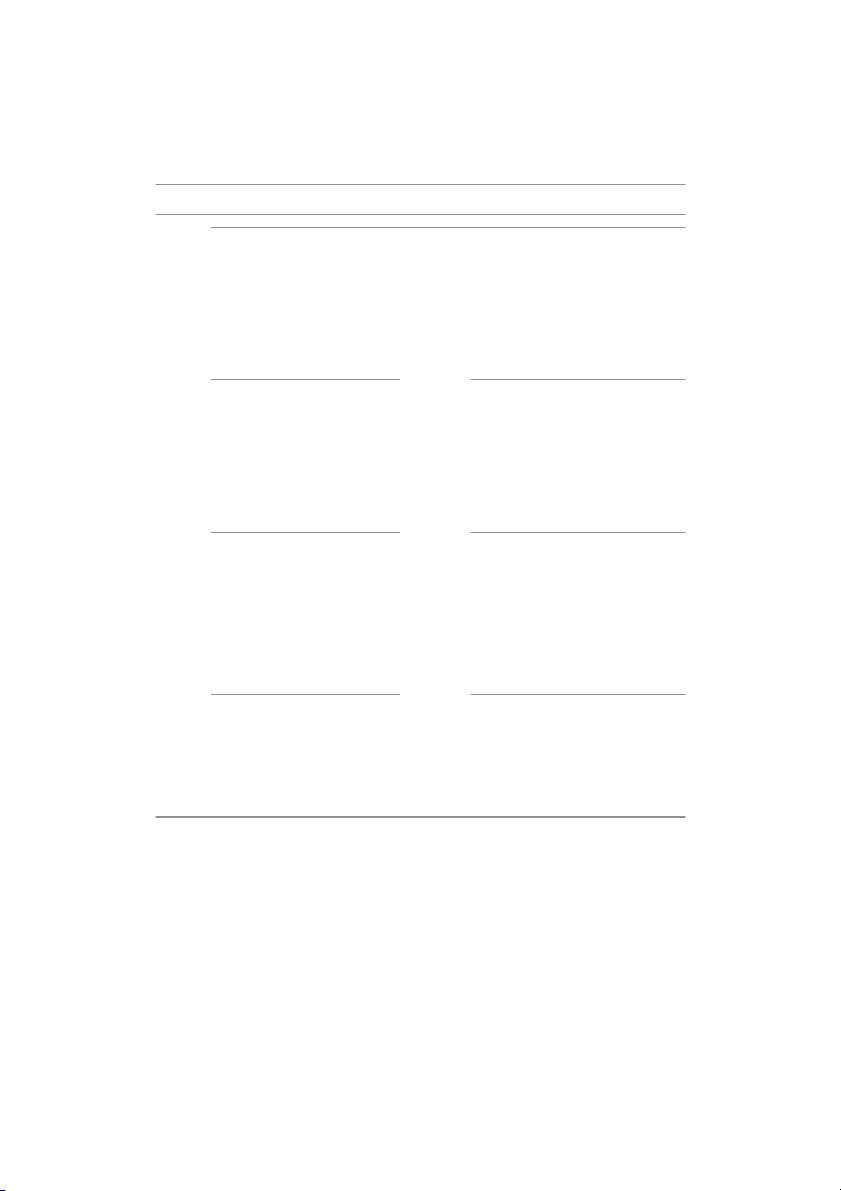
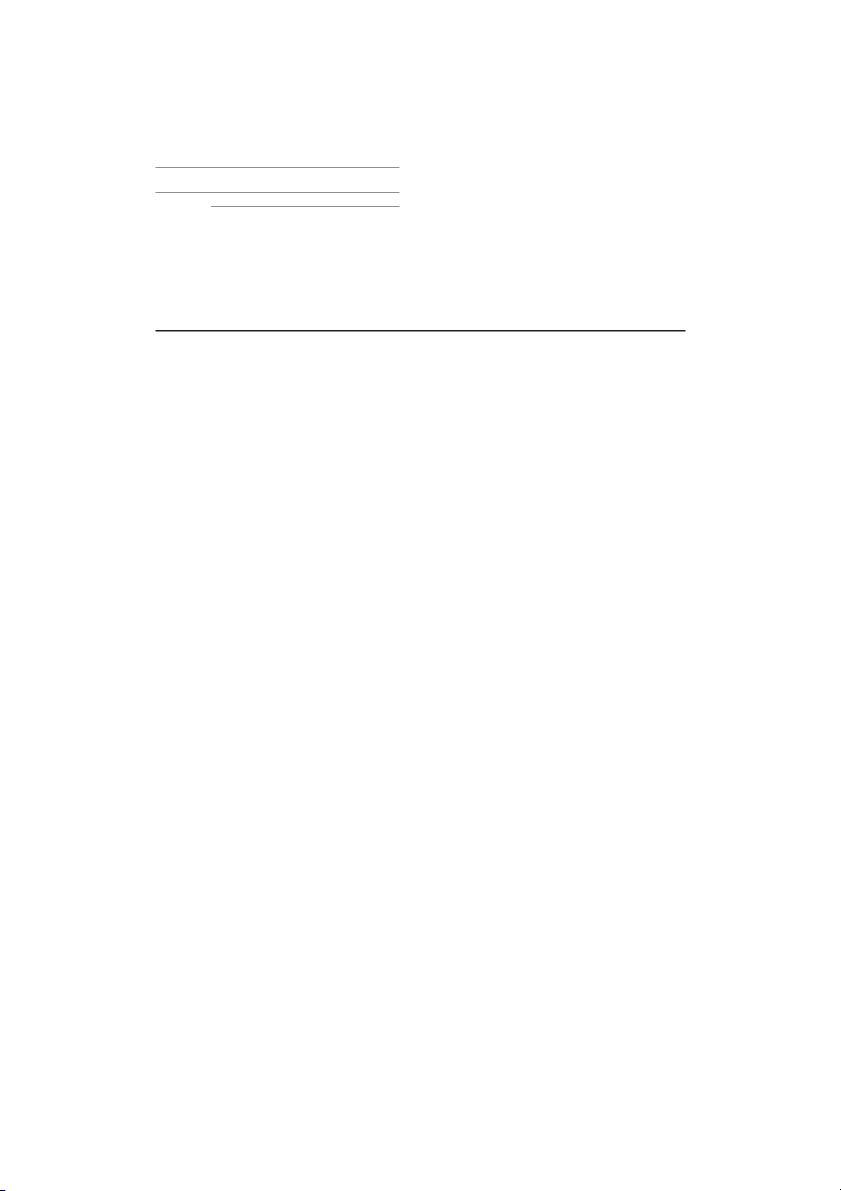
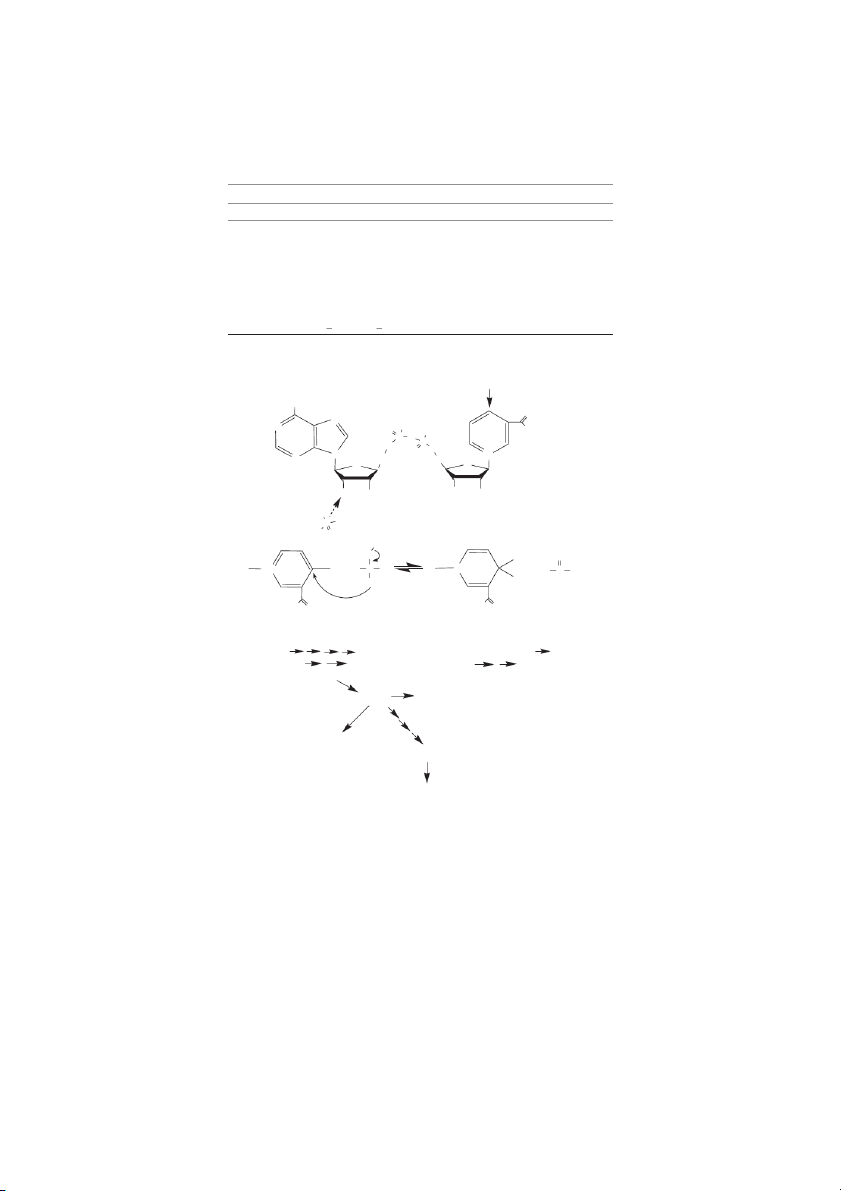
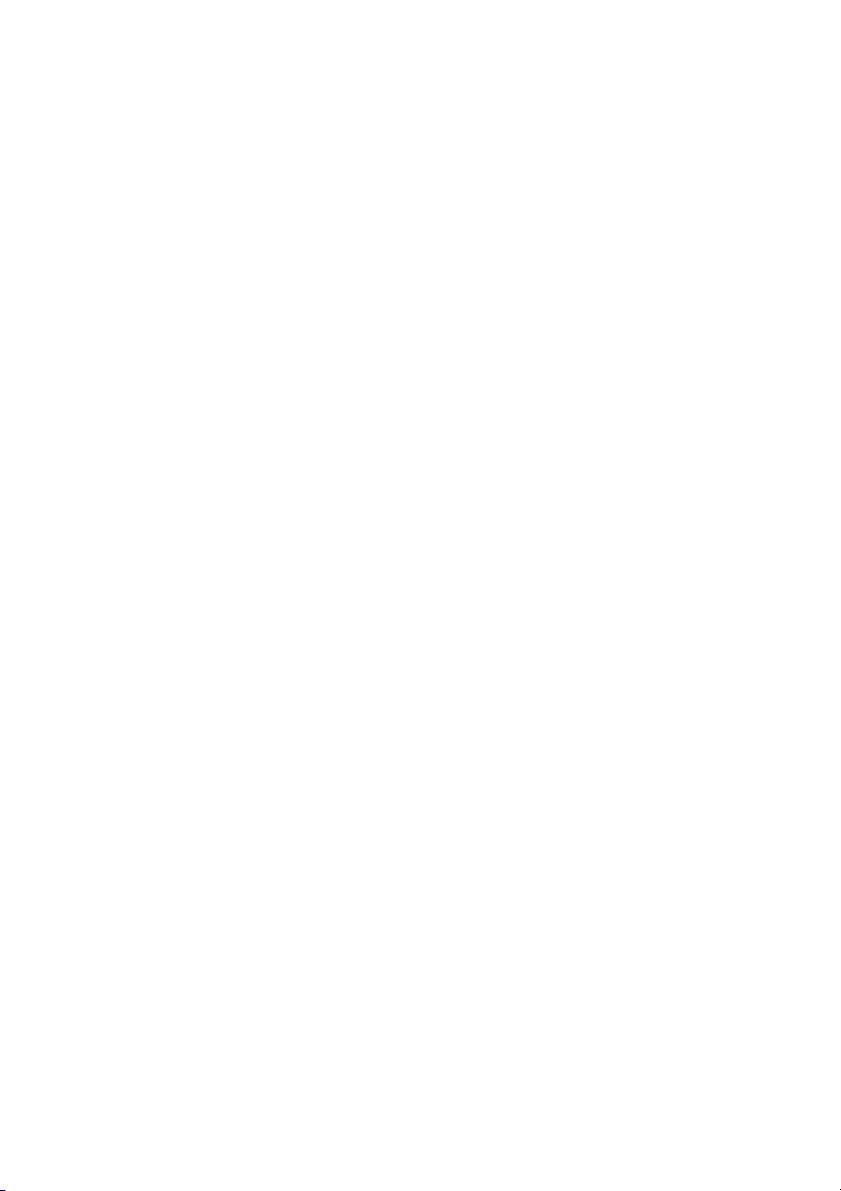
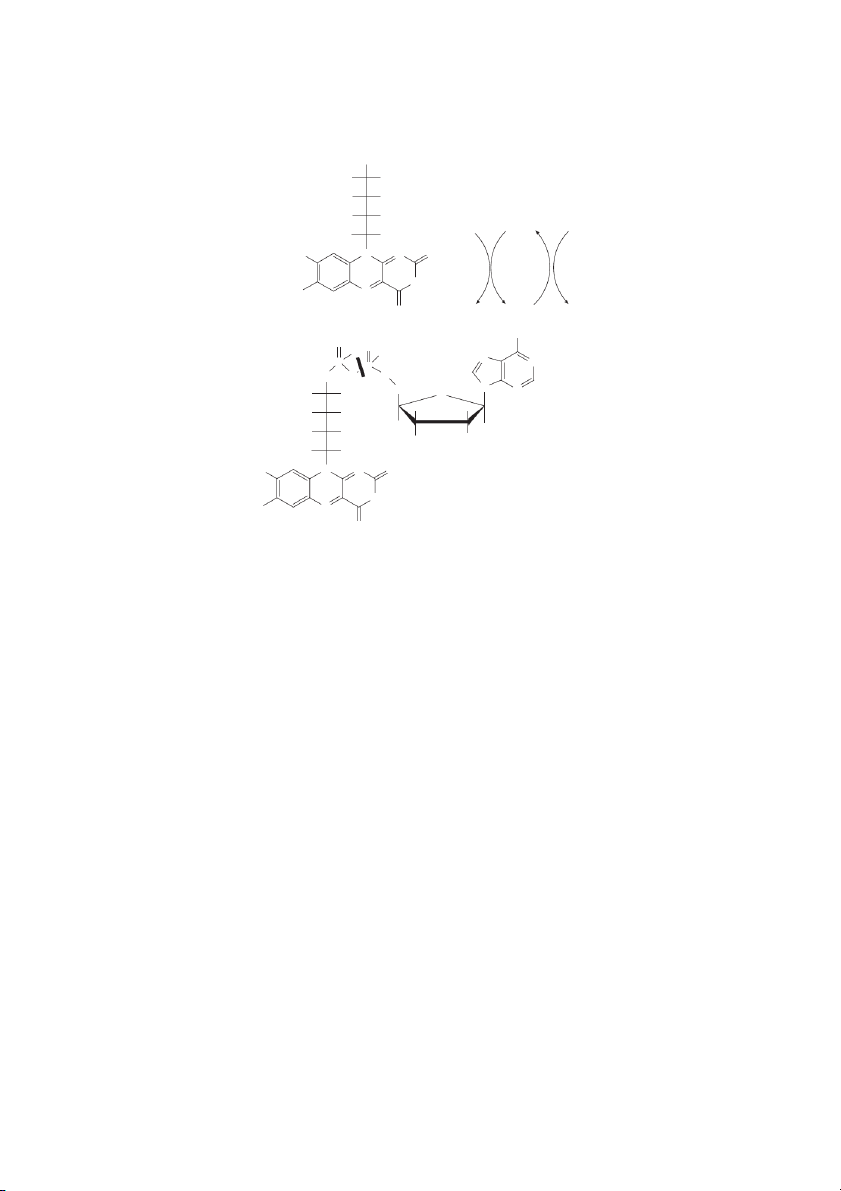
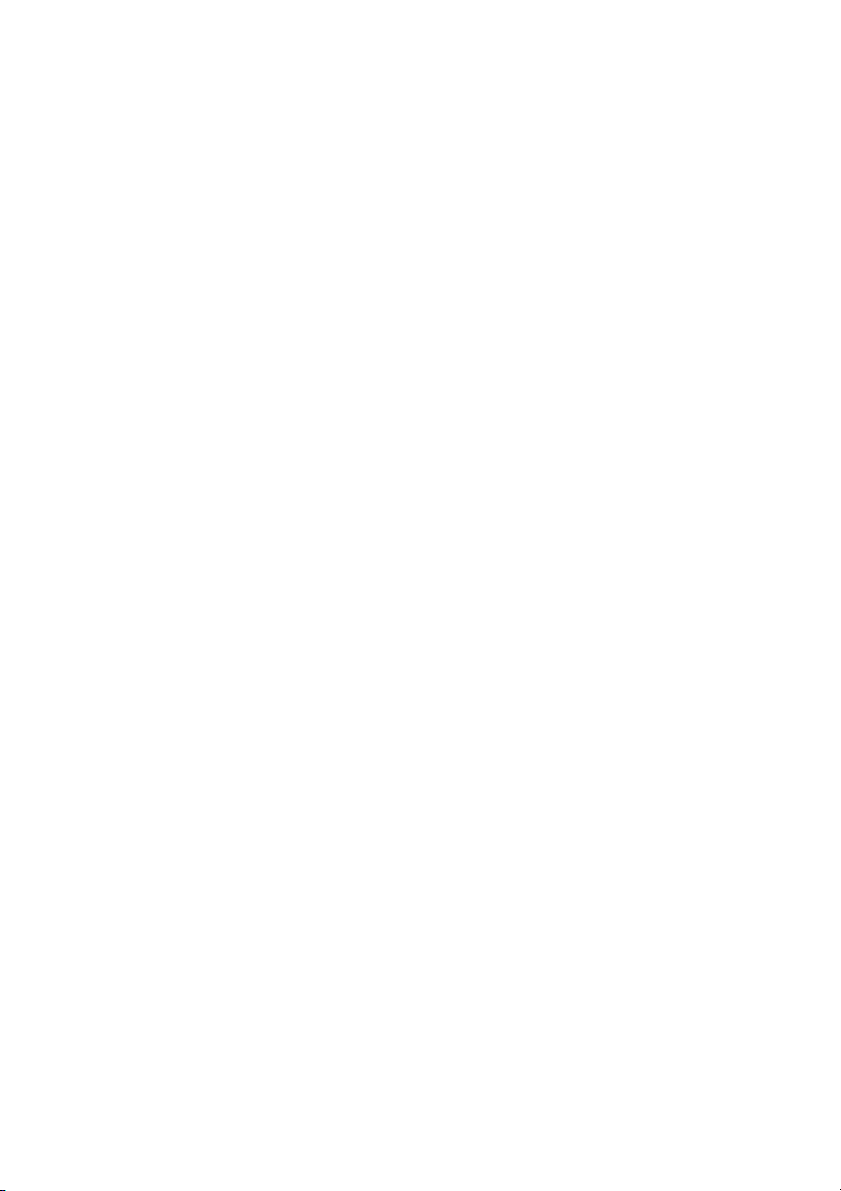
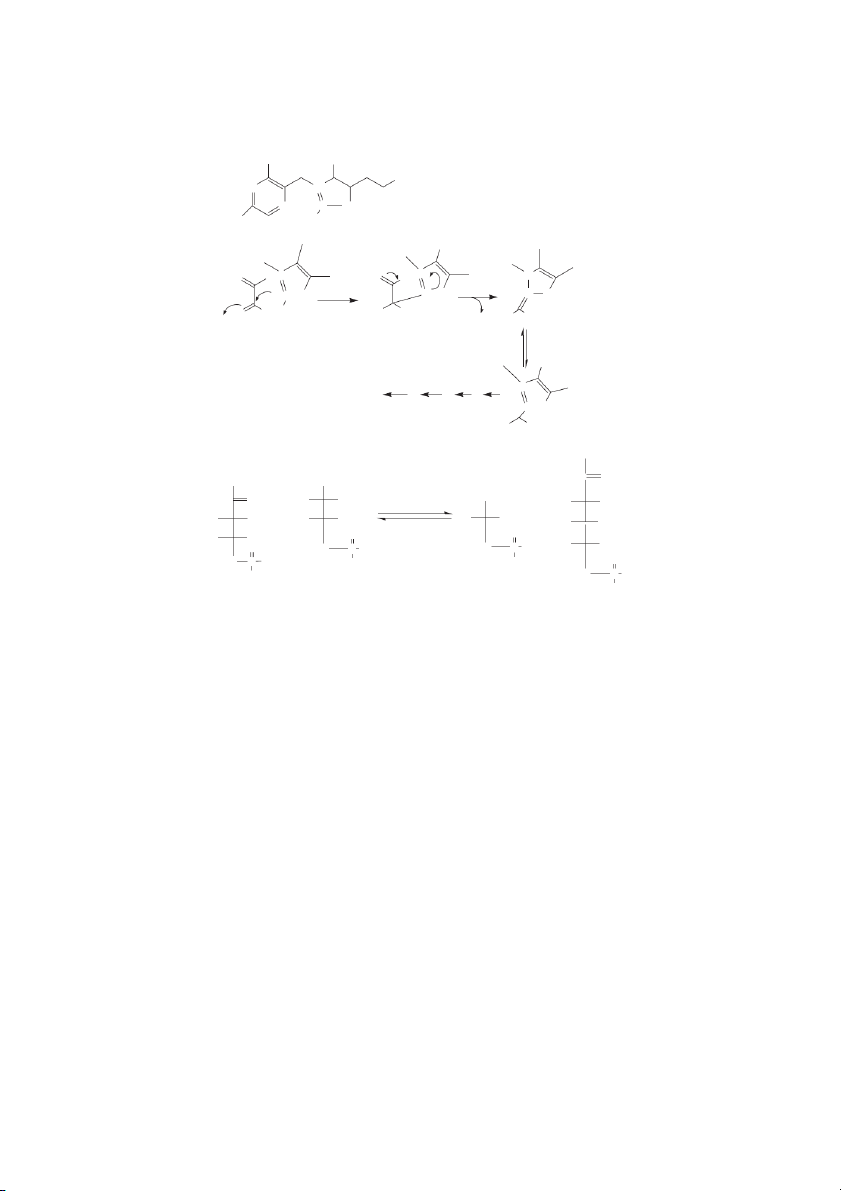
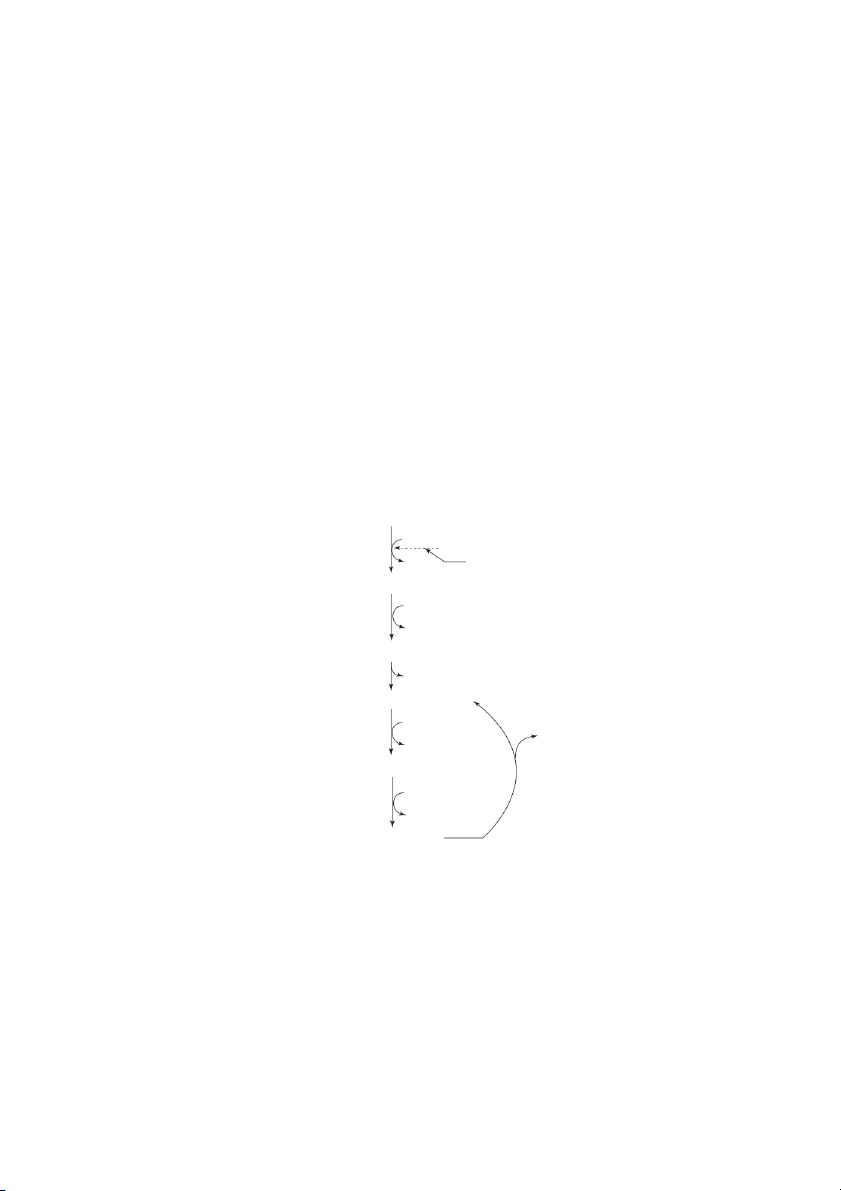
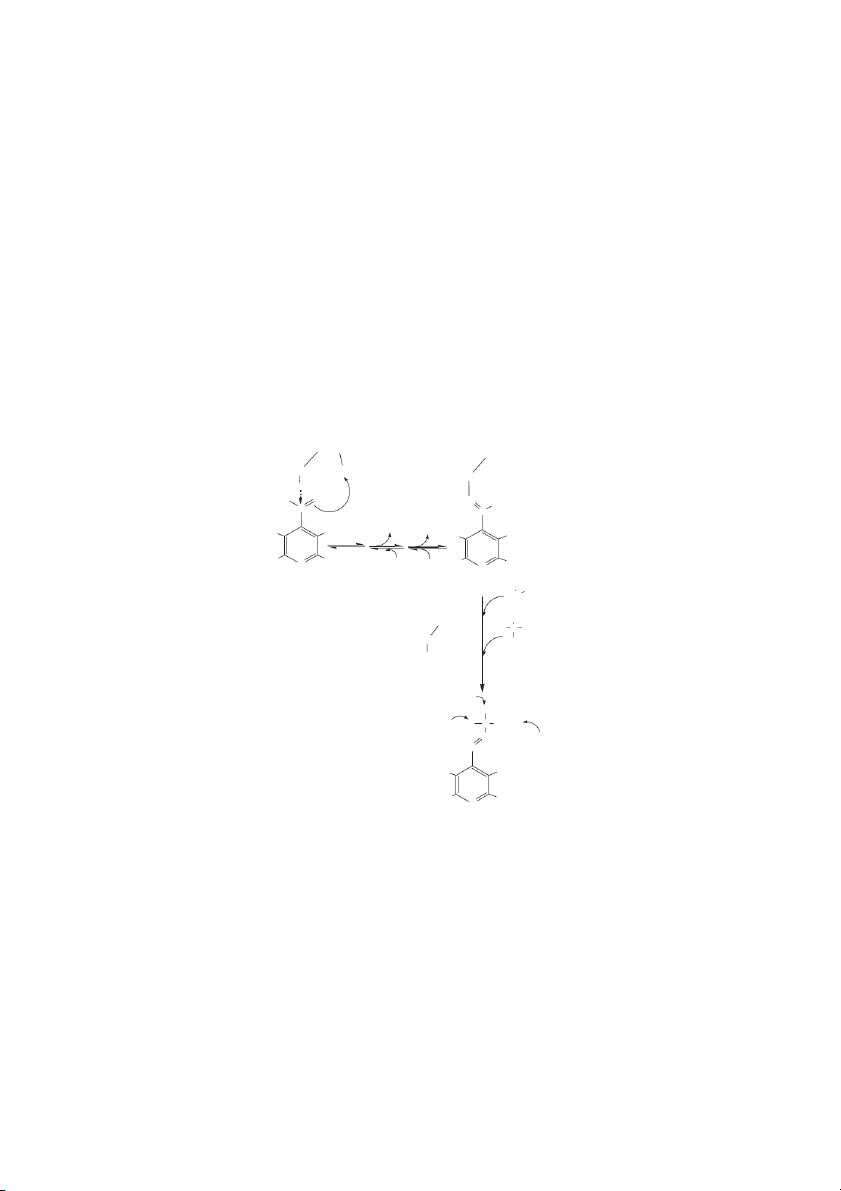
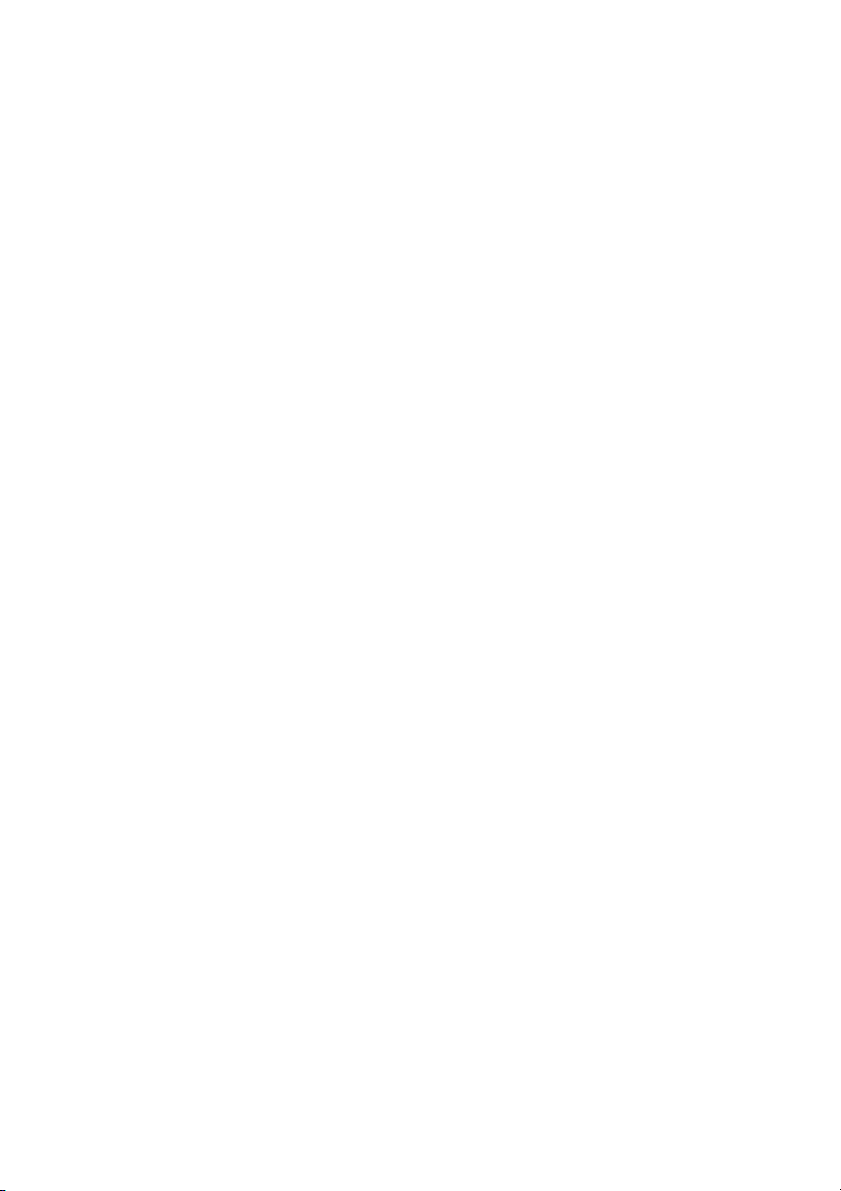
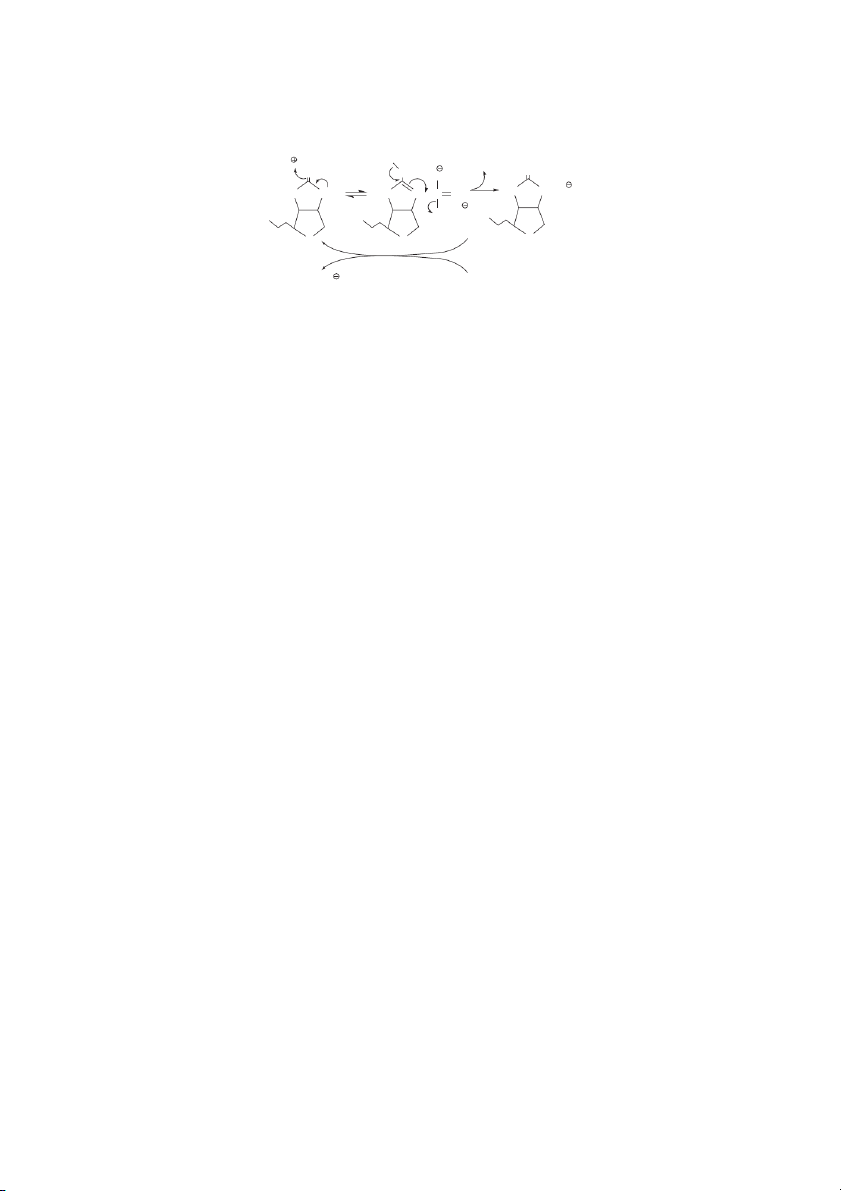
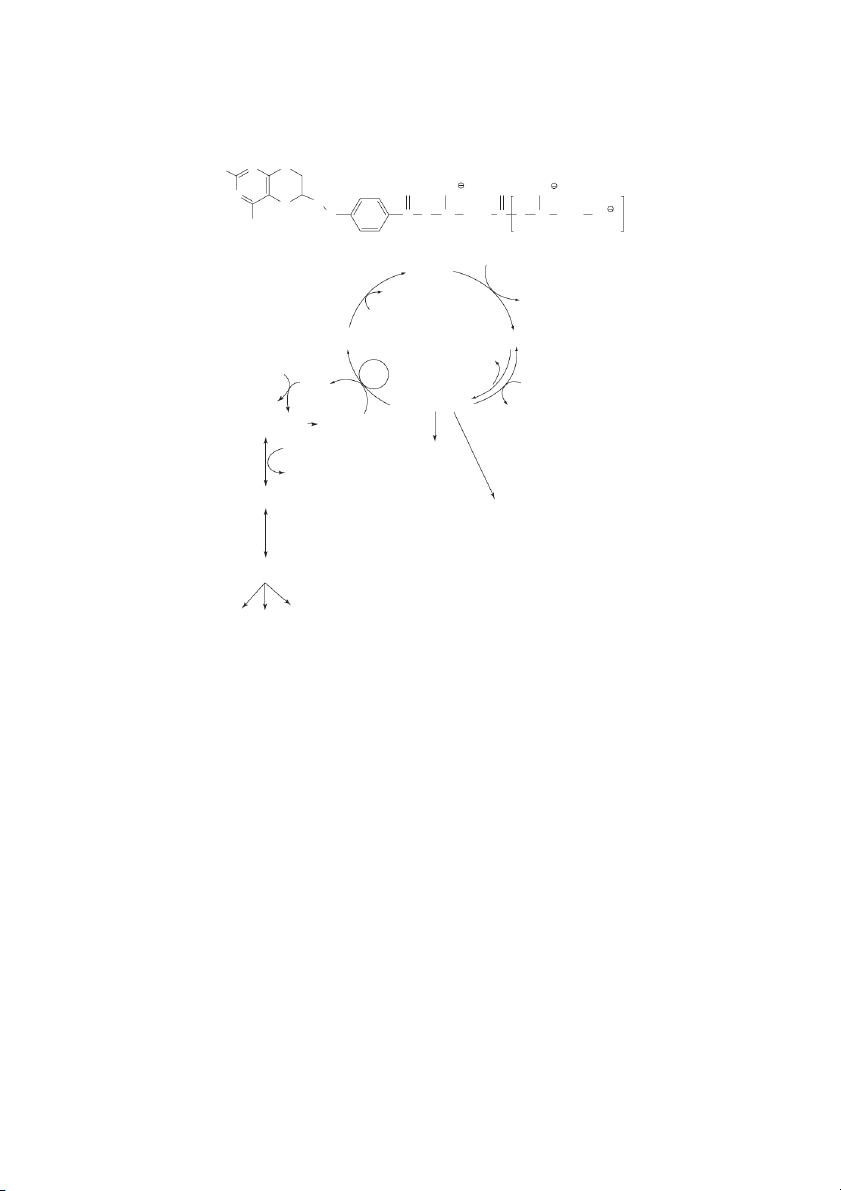
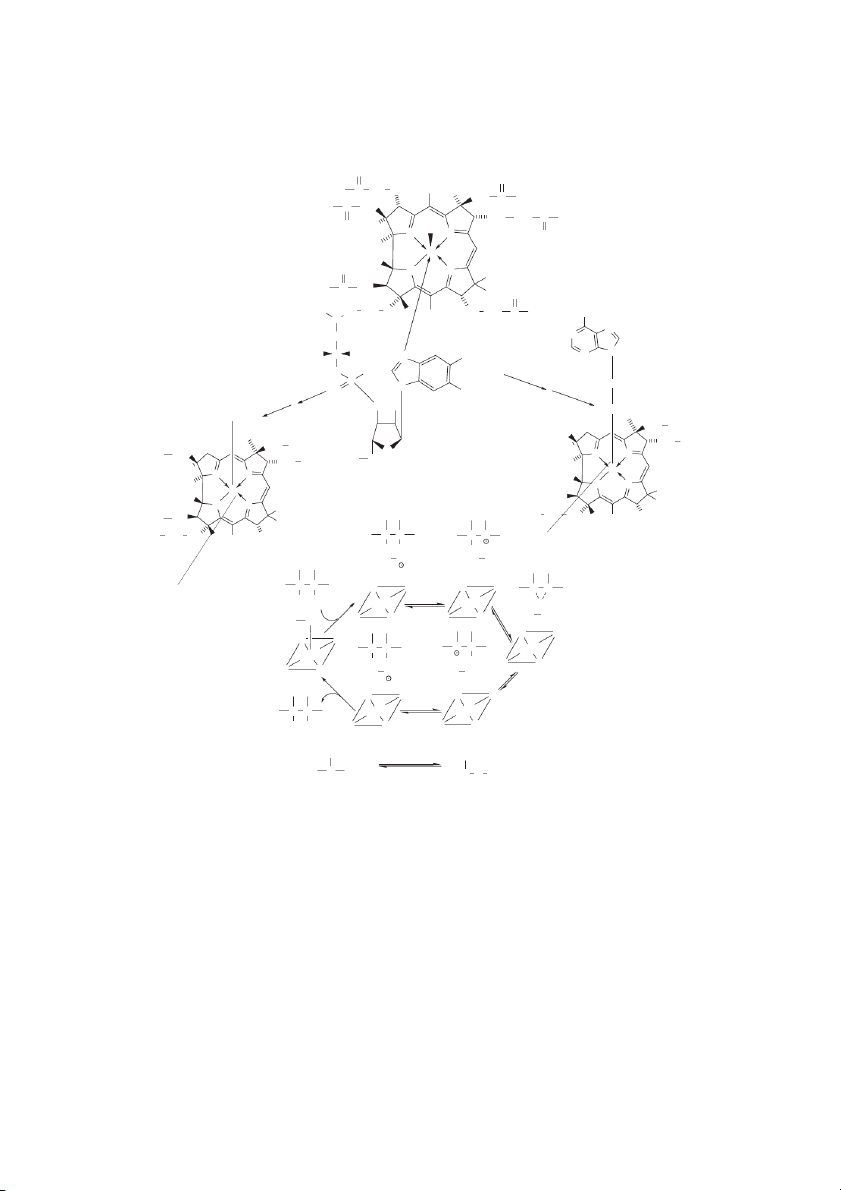
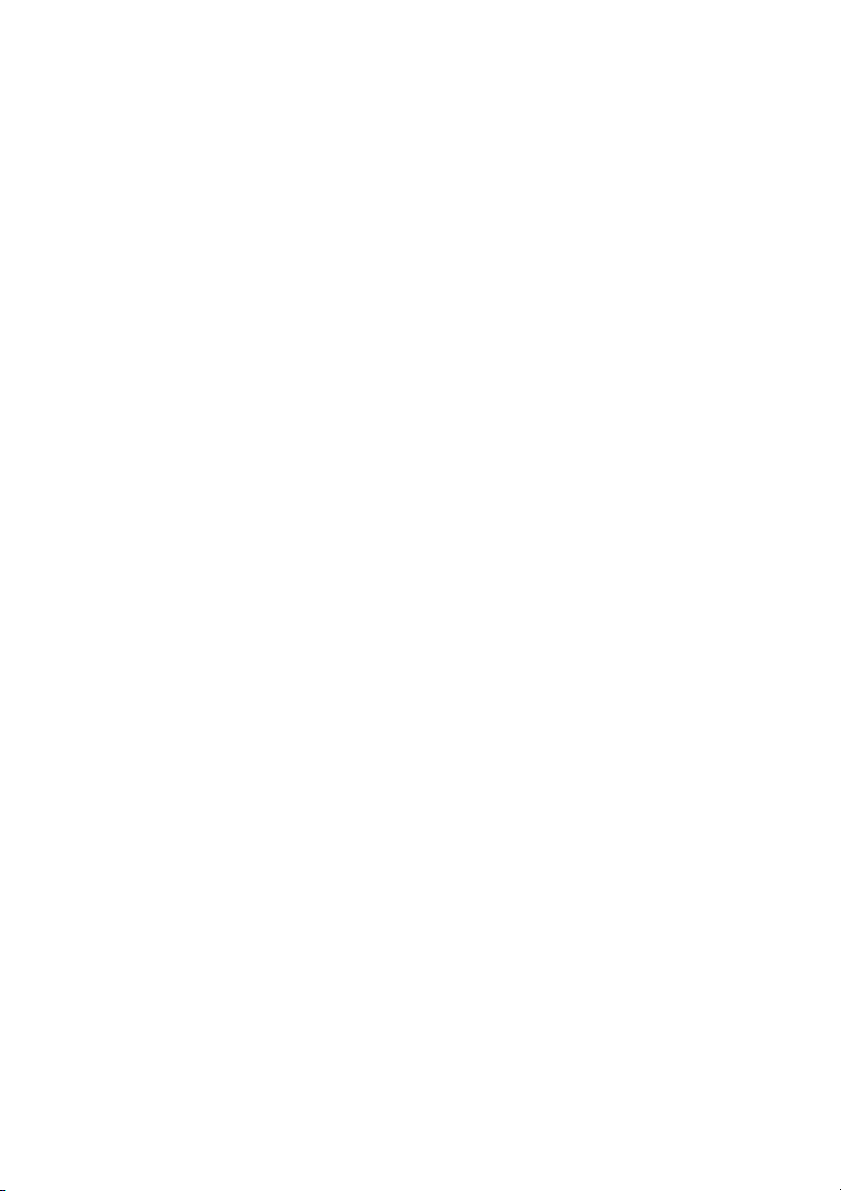
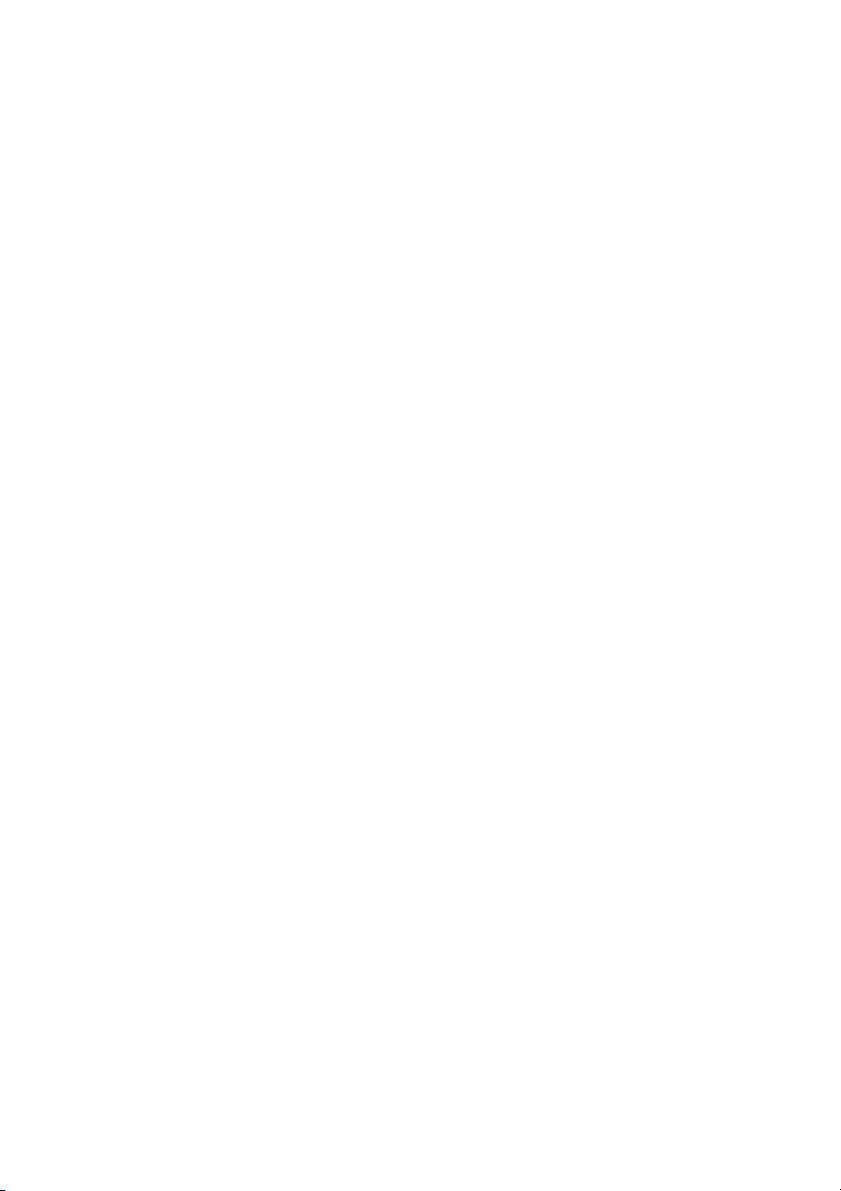
Preview text:
See discussions, stats, and author profiles for this publication at: https://www.researchgate.net/publication/339551721 0 The Water-Soluble Vitamins
Article · February 2020 CITATIONS READS 0 1,275 2 authors, including: Robert B Rucker
University of California, Davis
271 PUBLICATIONS8,002 CITATIONS SEE PROFILE
All content following this page was uploaded by Robert B Rucker on 28 February 2020.
The user has requested enhancement of the downloaded file. 10 The Water-Soluble Vitamins
Francene Steinberg and Robert B. Rucker
Department of Nutrition, UC Davis CONTENTS I.
Introduction ..........................................................................................................................................................10-2 II.
Vitamins Important in Redox Reactions ..............................................................................................................10-2 A.
Ascorbic Acid (Vitamin C)............................................................................................................................10-2 1.
Chemistry ..............................................................................................................................................10-2 2.
Absorption, Tissue Distribution, and Metabolic Functions ..................................................................10-2 3.
Requirement, Deficiency, and Toxicity ..................................................................................................10-3 4.
Food Sources and Stability ....................................................................................................................10-5 III.
The B Vitamins......................................................................................................................................................10-5 A.
Niacin ............................................................................................................................................................10-5 1.
Chemistry ..............................................................................................................................................10-5 2.
Absorption, Tissue Distribution, and Metabolism ................................................................................10-7 3.
Requirements, Toxicity, and Pharmacology ..........................................................................................10-7 4.
Food Sources and Stability ....................................................................................................................10-7 B.
Riboflavin ......................................................................................................................................................10-7 1.
Chemistry ..............................................................................................................................................10-7 2.
Absorption, Tissue Distribution, and Metabolism ................................................................................10-7 3.
Metabolism and Requirements ..............................................................................................................10-8 4.
Food Sources and Stability ....................................................................................................................10-8 IV.
B-Vitamins Important to Specific Features of Carbohydrate, Protein, or Lipid Metabolism ..............................10-9 A.
Thiamin..........................................................................................................................................................10-9 1.
Chemistry ..............................................................................................................................................10-9 2.
Absorption, Tissue Distribution, and Metabolism ................................................................................10-9 3.
Requirements, Pharmacology, and Toxicity ..........................................................................................10-9 4.
Food Sources and Stability ....................................................................................................................10-9 B.
Pantothenic Acid..........................................................................................................................................10-10 1.
Absorption, Tissue Distribution, and Metabolism ..............................................................................10-10 2.
Metabolic Functions and Requirements ..............................................................................................10-11 3.
Food Sources and Stability ..................................................................................................................10-11 C.
Pyridoxine ....................................................................................................................................................10-11 1.
Chemistry ............................................................................................................................................10-11 2.
Absorption, Tissue Distribution, and Metabolism ..............................................................................10-12 3.
Requirements, Pharmacology, and Toxicity ........................................................................................10-13 4.
Food Sources and Stability ..................................................................................................................10-13 V.
B-Vitamins Involved in Single Carbon and CO Transfer Reactions ................................................................10-13 2 A.
Biotin ..........................................................................................................................................................10-13 1.
Chemistry ............................................................................................................................................10-13 2.
Metabolism and Requirements ............................................................................................................10-13 3.
Food Sources and Stability ..................................................................................................................10-14 B.
Folic Acid and Vitamin B-12 ......................................................................................................................10-14 1.
Chemistry Folic Acid ..........................................................................................................................10-14 2.
Chemistry: Vitamin B-12 ....................................................................................................................10-14 3.
Digestion, Metabolism, and Tissue Distribution..................................................................................10-15 10-1 10-2
Handbook of Food Science, Technology, and Engineering, Volume 1 4.
Requirements, Pharmacology, and Toxicity ........................................................................................10-17 5.
Food Sources and Stability ..................................................................................................................10-17 VI.
Concluding Comments ......................................................................................................................................10-18
References ..................................................................................................................................................................10-18 I. INTRODUCTION
result in the acidity of ascorbic acid (pK = 4.2). When 1
ascorbic acid plays a role in reductions, the reaction usu-
The water-soluble vitamins consist of a mixed group of
ally occurs in a stepwise fashion with monodehydroascor-
chemical compounds. Their classification into specific
bic acid, as a semiquinone intermediate. This intermediate
chemical groups depends on both chemical characteristics
then disproportionates to ascorbic acid, and dehy-
and functions. The letter designations (vitamins B , B , 1 2
droascorbic acid (Figure 10.1).
B , etc., C) represent in part remnants from the past as the 3
discovery of given dietary growth or curative factors were given letter designations.
2. Absorption, Tissue Distribution, and
Vitamins are novel in their roles as “external” or Metabolic Functions
dietary regulatory agents. They have largely evolved to
Dietary ascorbic acid is absorbed from the duodenum and
serve: 1) specific cofactor and/or co-substrate functions,
proximal jejunum. Measurable, albeit small, amounts of
2) as regulatory agents, or 3) as antioxidants. All of the
ascorbic acid also cross the membranes of the mouth and
vitamins undergo specific and metabolically controlled
gastric mucosa. Although some controversy exists regard-
modifications before activation or conversion into their
ing the relationship between dietary intake and the intes-
functional forms. The most limiting events that control
tinal absorption of ascorbic acid, studies indicate that
function are often a specific step(s) in cofactor formation,
within the physiological ranges of intake (40–200 mil-
e.g., a phosphorylation reaction or ATP addition.
ligrams per day for humans or ~5–25 mg/kJ of diet);
The use of broad functional categories as headings to
80–90% of the vitamin is absorbed. Uptake at apical mem-
organize this chapter was chosen. For example, niacin,
branes involves a specialized Na-dependent, carrier-
riboflavin, and ascorbic acid serve primarily as redox
mediated system. Exit of ascorbic acid from enterocytes
cofactors. The roles of thiamin, pyridoxine (vitamin B-6)
utilizes a Na-dependent carrier system. To date, two Na+-
and pantothenic acid (as a component of coenzyme A) are
dependent L-ascorbic acid transporters have been cloned
distinguished because of their importance to carbohy-
and partially characterized. The preference for these trans-
drate, protein and amino acid, and acyl and acetyl trans-
porters is for L-ascorbic acid. Dehydroascorbic acid
port, respectively. Folic acid, vitamin B-12 (cobalamin),
uptake is facilitated by hexose transporters (3, 12).
and biotin will be discussed in relationship to their roles
In tissues, the highest concentration of ascorbic acid is
in single-carbon or CO transfer reactions. 2
found in the adrenal and pituitary glands followed by the
liver, thymus, brain, and pancreas. In diabetic animals, the
II. VITAMINS IMPORTANT IN REDOX
ascorbic acid content of tissue is often depressed, which REACTIONS
may be due to competition for uptake between dehydro
forms of ascorbic acid and glucose (3).
A. ASCORBIC ACID (VITAMIN C)
Ascorbic acid is maintained in cells by several mech-
Ascorbic acid functions primarily as a cofactor for micro-
anisms. Ascorbic acid reductases maintain L-ascorbic
somal mono-and dioxygenases (hydroxylases) and oxi-
acid in the reduced form, which is less susceptible to easy
dases. In most animals, ascorbic acid is synthesized from
diffusion. Within most cells, measurable amounts of
glucose in the liver. In birds and reptiles, ascorbic acid
ascorbic acid are also maintained as the 2-sulfate deriva-
tive. The ability to maintain ascorbic acid in the reduced
synthesis takes place primarily in the kidney. In animals
state and as ascorbic acid-2-sulfate appear important in
that require ascorbic acid, e.g., humans, it is a deficiency
maintaining cellular ascorbic acid levels (12).
of gulonolactone oxidase, the last step in ascorbic acid
In the neonate, glutathione is also very important to
synthesis, which results in the need for a dietary source
ascorbic acid recycling. In this regard, an argument can be (Figure 10.1; 12).
made for a dietary need for ascorbic acid in the neonates
of some animal species in which the adult does not have a 1. Chemistry
dietary requirement. For example, the levels of glu-
Ascorbic acid (2,3-enedial-glulonic acid) is a powerful
tathione are relatively low in neonate rat and mouse tis-
cellular reducing agent and is of general importance as an
sues. As ascorbic acid is oxidized to dehydroascorbic
antioxidant because of its high reducing potential. Both of
acid, there is the need for conservation, because dehy-
the hydrogens of the enediol group dissociate, which
droascorbic acid is easily degraded. Glutathione in its The Water-Soluble Vitamins 10-3 O O K 10 a1 = 8⋅ −5 D-glucose − H+ O O O O H H (−) O O K ⋅ −12 OH OH a2= 1.6 10 H H H H H H H Glulonolactone OH OH Ascorbic acid Superoxide Hydrogen peroxide GSSG ROS and oxyl Monodehydroascorbate radicals (e.g., reductase tocopheroxyl Dehydroascorbate radical) reductase Monodehydroascorbate 2 GSH O O O O OH H H H Oxalate + Tartrate OH or Dehydroascorbate CO2 + C metabolites 5
FIGURE 10.1 Ascorbic acid. In most animals ascorbic acid is derived from glucose. Gulonolactone is produced from glucose and
its oxidation to ascorbic acid is catalyzed by gulonolactone oxidase (dashed line). The K s for the two dissociable protons in ascor- a
bic acid are indicated. At pH 7, dehydroascorbic acid can be reduced to ascorbic acid with a Eo = 0.08, e.g., dehydroascorbate
2e 2H ↔ ascorbate. In a biological system, reduction of the monodehydroascorbic acid to ascorbic acid occurs by the NADH-
requiring enzyme, monodehydroascorbic acid reductase. Reduction of dehydroascorbic acid utilizes reduced glutathione and is cat-
alyzed dehydroascorbic acid reductase, also known as glutaredoxin.
reduced form serves as the reducing substrate for glutare-
(at specific prolyl and lysyl residues). This can result in
doxin, an enzyme that can maintain ascorbic acid in a
abnormal collagen degradation and, as a consequence, reduced state (3, 12).
decreased collagen production. In addition, the inability to
As a cellular reducing agent, ascorbic acid plays a
deal with metabolic stress requiring normal adrenal gland
number of very important roles. It serves as a cofactor for
function and abnormal fatty acid metabolism (carnitine
mixed-function oxidations that result in the incorporation
synthesis) can be signs of scurvy.
of molecular oxygen into various substrates. Examples
The current requirement for humans is 75 mg per day
include the hydroxylation of proline in collagen, elastin,
(U.S. RDA for the healthy adults) or 30–60 mg per 1000
C1q complement, and acetylcholine esterase. Some of the
kcal or 4 MJ of diet (4; Table 10.1). Because of the mech-
P-450-dependent hydroxylases that carry out the hydrox-
anisms in place to homeostatically regulate ascorbic acid,
ylation of steroids, drugs, and other xenobiotics also have
evidence of toxicity, other than gastric upset, is seldom
a need for ascorbic acid (12).
reported (e.g., diarrhea, gastric irritation). Evidence of
Ascorbic acid-dependent hydroxylation steps also
toxicity, however, may be manifest, when ascorbic acid is
occur in the biosynthesis of carnitine and the hydroxyla-
consumed in gram quantities per day. Of interest, ascorbic
tion of tyrosine in the formation of catecholamines. Most
acid intake in this range can result in decreased histamine
of the enzymes involved in these processes are metal-
production and facilitate reduction of nitrosamines and
requiring enzymes, in which the role of ascorbic acid is to
other putative cancer promoting agents with similar
maintain the metal (usually Cu or Fe) in a reduced state. chemical characteristics. The interaction with
nitrosamines occurs primarily in the stomach, and is
chemical in nature owing to ascorbic acid’s potential to
3. Requirement, Deficiency, and Toxicity
function as an excellent reducing agent. The anti-hista-
Most animals with the exception of primates, guinea pigs,
mine effects from pharmacologic exposure of ascorbic
some snakes, fruit-eating bats, birds such as passerines,
acid are in part the basis for its use as an analgesic in the
and salmonid fish synthesize ascorbate (Figure 10.1).
treatment of “common colds.”
Impaired collagen synthesis is a principle feature of ascor-
When fed in excess of metabolic need, tissue levels of
bic acid deficiency, which is evidenced in capillary
ascorbic acid are homeostatically maintained. Homeostasis
fragility, bleeding gums, delayed wound healing, and
occurs by the induction of ascorbic acid decarboxylases,
impaired bone formation. Connective tissue lesions are
which initiates enhanced degradation of ascorbate to CO2
primarily a result of under-hydroxylated collagen and C-4 or C-5 fragments (12). 10-4
Handbook of Food Science, Technology, and Engineering, Volume 1 TABLE 10.1
The Daily Reference Intakes for the Water-Soluble Vitamins (Part 1)a Age Age Vitamin Condition EARb RDAc AId ULe Vitamin Condition EAR RDA AI UL Ascorbic Years mg/day mg/day mg/day mg/day Niacin Years mg/day mg/day mg/day mg/day Acid 0–0.5 – – 40 – 0–0.5 – – 2. – 0.5–1 – – 50 – 0.5–1 – – 4. – 1–3 13 15 – 400 1–3 5 6 – 10 4–8 22 25 – 650 4–8 6 8 – 15 9–13 39 45 – 1200 9–13 9 12 – 20 14–18 63 75 – 1800 14–18 12 14 (M), 16 (F) – 30 19–>70 75 90 – 2000 19–>70 11 14 (M), 16 (F) – 35 19–30 70 85 – 2000 19–30 13 17 – 35 Pregnancy Pregnancy 19–30 100 120 – 2000 19–30 13 17 – 35 Lactation Lactation Ribofla- Years mg/day mg/day mg/day mg/day Thiamin Years mg/day mg/day mg/day mg/day vin 0–0.5 – – 0.3 – 0–0.5 – – 0.2 0.5–1 – – 0.4 – 0.5–1 – – 0.3 1–3 0.4 0.5 – – 1–3 0.4 0.5 – 4–8 0.5 0.6 – – 4–8 0.5 0.6 – 9–13 0.8 0.9 – – 9–13 0.7 0.9 – 14–>70 0.9 (M), 1.1 (M), – – 14–>70 0.9 (M), 1.1 (M), – 1.1 (F) 1.3 (F) 1.0 (F) 1.2 (F) 19–30 1.2 1.4 – – 19–30 1.2 1.4 – Pregnancy Pregnancy 19–30 1.3 1.6 – – 19–30 1.2 1.5 – Lactation Lactation Panto-thenic Years mg/day mg/day mg/day mg/day Vitamin Years mg/day mg/day mg/day mg/day Acid 0–0.5 – – 1.7 – B-6 0–0.5 0–0.5 – – 0.1 0.5–1 – – 1.8 – 0.5–1 0.5–1 – – 0.3 1–3 – – 2 – 1–3 0.4 0.5 – 30 4–8 – – 3 – 4–8 0.5 0.6 – 40 9–13 – – 4 – 9–13 0.8 1.0 – 60 14–>70 – – 5 – 14–>70 1.1 1.3 – 80 19–30 – – 6 – 19–30 1.1 1.3 – 100 Pregnancy Pregnancy 19–30 – – 7 – 19–30 1.1 1.3 – 100 Lactation Lactation
The Daily Reference Intakes for the Water-Soluble Vitamins (Part 2)a Biotin Years μg/day μg/day μg/day μg/day Folic Years μg/day μg/day μg/day μg/day 0–0.5 – – 5.0 – Acid 0–0.5 – – 65 – 0.5–1 – – 6.0 – 0.5–1 – – 80 – 1–3 – – 8.0 – 1–3 120 150 – 300 4–8 – – 12 – 4–8 160 200 – 400 9–13 – – 20 – 9–13 320 400 – 1000 14–>70 – – 25 – 14–>70 320 400 – 1000 19–30 – – 30 – 19–30 520 600 – 1000 Pregnancy Pregnancy 19–30 – – 30 – 19–30 520 600 – 1000 Lactation Lactation (Continued) The Water-Soluble Vitamins 10-5 TABLE 10.1 (Continued ) Age Vitamin Condition EARb RDAc AId ULe Vitamin Years μg/day μg/day μg/day μg/day B-12 0–0.5 – – 0.4 – 0.5–1 – – 0.5 – 1–3 0.7 0.9 – 4–8 1 1.2 – – 9–13 1.5 1.8 – – 14–>70 2 2.4 – – 19–30 2.2 2.6 – Pregnancy 19–30 2.2 2.6 - – Lactation
a Dietary Reference Intakes (DRI) — Reference values that are quantitative estimates of nutrient intakes to be used for planning and assessing diets
for apparently healthy people. There are 4 reference values: the EARs, RDAs, AIs, and UIs.
b Estimated Average Requirement (EAR) — The nutrient intake value that is estimated to meet the requirement of half of the healthy individuals in a
group. The EAR is used to assess the adequacy of dietary intakes within a population and it is used to develop the RDAs.
c Recommended Dietary Allowances (RDA) — The average daily dietary intake level that is sufficient to meet the nutrient requirements of nearly all
(97–98%) healthy individuals in a designated group.
d Adequate Intake (AI) — AI is used when the RDA for a nutrient is not available. It is a recommended daily intake that is based on observed or exper-
imentally determined approximations of nutrient intake by a group of healthy people. An AI is developed when there is no EAR or research to help
develop the RDA for a nutrient.
e Tolerable Upper Intake Level (UL) — Highest level of daily nutrient intake that is likely to pose no risks of adverse health effects to almost all indi-
viduals in the general population.
4. Food Sources and Stability
became associated with the vitamin, niacin, and the con-
sumption of corn (maize), as a principle source of dietary
Ascorbic acid is widely distributed in fruits and vegeta-
energy. The nutritional availability of niacin in corn is rel-
bles, with foods such as citrus fruits, berries, kiwi, pep-
atively low bioavailability. Corn also has a low content of
pers, Brussels sprouts, and broccoli being particularly
tryptophan. As shown in Figure 10.2, niacin can be gener-
high in this vitamin. Ascorbic acid is relatively unstable
ated upon tryptophan degradation.
and easily destroyed during processing and storage of
Normally, niacin is derived from foods, such as corn,
foods. It is labile to neutral and alkaline conditions, heat,
by hydrolysis of NAD (nicotinamide adenosyl dinu-
light, and exposure to oxygen. The rate of decomposition
cleotide) and NADP (nicotinamide adenosyl phosphodi-
is accelerated by the presence of metals, especially iron
nucleotide) by the action of pancreatic or intestinal nucle-
and copper, and by enzymes such as peroxidases. Food
osides and phosphatases. As most human diets now con-
preservation techniques that act to limit losses of ascorbic
tain adequate tryptophan, and available NAD and NADP,
acid are blanching of vegetables, pasteurization, and
niacin deficiency is seldom observed (6).
deaereation of juices to limit oxygen and inactivate the
enzymes. Additionally, packaging in containers that limit 1. Chemistry
exposure to light and oxygen will maintain ascorbate con-
centrations. Erythorbic acid, a biologically inactive ana-
NAD and its phosphorylated form, NADP, are two coen-
log of ascorbic acid, is often added to foods as an
zymes derived from niacin. Both contain an unsubstituted
antioxidant to preserve freshness. A summary of chemical
pyridine 3-carboxamide that is essential to function in
characteristics for ascorbic acid and other B-vitamins is
redox reactions with a chemical potential near –0.32 V. given in Table 10.2 (4, 13).
Virtually all cells are capable of converting niacin to
NAD. Most enzymes that require NAD are oxidoreduc- tases (dehydrogenases). III. THE B VITAMINS
NAD catalyzes a diverse array of reactions, such as A. N
the conversion of alcohols and polyols to aldehydes or IACIN
ketones. The most common mechanisms involve the
Throughout the 18th and 19th centuries, the disease pella-
sterospecific abstraction of a hydride ion [H: (-)] from the
gra was prevalent in Western Europe and the southern
substrate with its subsequent transfer. It is of interest that
region of the United States. This disease would eventually
cells generally delegate NAD to enzymes in catabolic 10-6
Handbook of Food Science, Technology, and Engineering, Volume 1 TABLE 10.2
Stability of Water-Soluble Vitamins Effect of pH Vitamin Acid Neutral Alkaline Oxygen UV Light Heat Ascorbic Acid S U U U U U Biotin S S U S S S Cobalamin (B-12) S S S U U S Folic acid S U U U U U Niacin S S S S S S Pantothenic acid U S U S S U Pyridoxine (B-6) S S S S U U Riboflavin (B-2) S S U S U S Thiamin (B-1) S S U U S U S=Stable, U=Unstable. NH2 O N N OH O OH NH P 2 O P O O O N N+ N H2C H O 2C O OH OH OH OH HO P OH HO O H X H X R R +N H R C R R N + R C R + H+ + H HS H H 2N O 2N O Tryptophan
-amino--carboxymuconic- -semialdehyde Quinolinic acid Nicotinic acid mononucleotide Nicotinic Acid dinucleotide NAD NADP ADP-ribose poly(ADP-ribose) mono(ADP-ribose) Nicotinamide cyclic ADP-ribose N-methylnicotinamide
FIGURE 10.2 NAD and NADP. The structures for NAD and NADP (via ATP-derived phosphate addition to NAD, cf. dashed arrow)
and their site of hydrogen transfer (solid arrow) are indicated along with a mechanism of hydrogen transfer. The HR and HS desig-
nations are shown to indicate that the transfer occurs with stereospecificity. NAD and NADP are products of tryptophan degradation
through the quinolate portion of the tryptophan degradation pathway. In addition to acting as a reductant, NAD is precursor to ribo-
sylations and cyclic ribosylated products that are important to cellular regulation. The Water-Soluble Vitamins 10-7
pathways, whereas NADP is utilized in synthetic path- B. RIBOFLAVIN
ways. An additional and equally important function of
NAD is its role as a substrate in poly- and monoribosyla-
Riboflavin was one of the first of the B vitamins identified
tion reactions. Mono and polyribosylation post-transla-
(10). Originally, it was thought to be the heat stable factor
tional chemical modifications are important in many
responsible for the prevention of pellagra. Riboflavin is
cellular regulatory functions (see next section).
present in tissue and cells as FAD (flavin adenine dinu-
cleotide) and FMN (flavin adenine mononucleotide). FAD
and FMN are cofactors in aerobic processes, usually as
2. Absorption, Tissue Distribution, and
cofactors for oxidases, although FAD can also function in Metabolism
anaerobic environments as a dehydrogenase cofactor.
Transporters for niacin uptake have been identified, but are
not as well characterized as some of the other vitamin 1. Chemistry
transporters. As noted, NAD may be derived from trypto-
Flavins, such as riboflavin derivatives (Figure 10.3), are
phan through the so-called quinolinate pathway (Figure
ideally suited to catalyze one electron, one-proton transfer
10.1). About half the niacin as NAD or NADP is associated
reactions. Oxygen is often utilized as a co-substrate in the
with enzymes; the remainder is available as a substrate for
redox reactions catalyzed by FAD- and FMN-containing
mono- and polyribosylation reactions that are important in
enzymes. Carbon-carbon double bond formation and
the regulation of a broad range of enzymes. In the nuclei of
redox of sulfur-containing compounds, e.g., lipoic acid,
cells, polyribosylation of specific histones precedes the
are catalyzed also by flavoproteins. NADP(H) H com-
normal process of DNA repair. When niacin is in excess,
monly serves as a reductant in processes to regenerate
most mammals convert it to N-methylnicotinamide, which
FAD and FMN. Riboflavin has a number of designations,
has a low renal threshold and is excreted (6).
some of which are still in use (vitamin B-2, vitamin G,
ovoflavin, uroflavin, lactoflavin, heptoflavin).
3. Requirements, Toxicity, and Pharmacology
Riboflavin was separated and identified from other B
Niacin is needed in amounts corresponding to 14 to 16 mg
vitamins, because it is heat stable, but light instable and
per day or 4–6 mg per 1000 kcal or 4 MJ of diet (4; Table
fluorescent. The chemical name for riboflavin is 6,7-
10.1). Niacin requirements are often expressed as equiva-
dimethyl-9-(d-1-ribityl) isoalloxazine. Deviations in
lents, where one equivalent corresponds to 1 mg of niacin.
structure can markedly affect activity (10). For example,
The conversion of tryptophan to niacin produces about
the polyol side-chain must be in the D-form and substitu-
1 mg or equivalent of niacin for every 60 mg of trypto-
tion in other positions causes loss of activity or gives rise phan degraded (Figure 10.2).
to compounds that have inhibitory activity, e.g.,
There are a number of therapeutic uses for pharmaco- 5,6-dimethyl (isoriboflavin).
logic doses of niacin-derived compounds, when increased
blood flow is desirable. Nicotinic acid can cause vasodi-
2. Absorption, Tissue Distribution, and
latation when consumed in amounts of 100 mg or more. Metabolism
Niacin in gram quantities is an effective lipid-lowering
Like most water-soluble vitamins, riboflavin is absorbed
agent (increases HDL). However, in some individuals,
flushing, gastric irritation, and other similar side effects
in the proximal part of the small intestine by a carrier-
caused by pharmacologic doses of nicotinic acid or niacin
mediated process. Riboflavin is not particularly soluble. may preclude use (6).
There are upper limits to riboflavin’s availability, which
are dictated in part by solubility. In this regard, an impor-
tant chemical characteristic that is attributed to the ribose
4. Food Sources and Stability
moiety is improved water solubility. Isoalloxazines are
Niacin is found in high levels in animal tissues (chicken,
not particularly water-soluble.
fish, beef – especially liver), and peanuts, and in moderate
Intestinal riboflavin uptake is adaptively regulated by
amounts in whole grains such as wheat and barley,
dietary levels, and by specific intracellular protein kinase-
enriched cereal products, mushrooms, and some vegeta-
mediated regulatory pathways. Over-supplementation
bles such as corn and peas. Niacin is very stable in a vari-
with pharmacological amounts of riboflavin can lead to a
ety of conditions. Indeed some treatments such as
significant and specific down-regulation in riboflavin
moderate heat and alkali treatment of corn can result in
uptake; riboflavin deficiency causes a significant and spe-
greater niacin availability (Table 10.2). An excess of water
cific up-regulation in intestinal riboflavin uptake. Factors
used in cooking and processing of foods can result in
that have been shown to interfere with the normal intes-
leaching of the vitamin, as is similar for all water-soluble
tinal riboflavin uptake process include alcohol and certain vitamins (4).
tricyclic drugs (e.g., chlorpromazine). 10-8
Handbook of Food Science, Technology, and Engineering, Volume 1 CH2OH HO H
Reductive and oxidative half-reactions HO H of flavins in proteins HO H Riboflavin Fl BH ox 2 H H AH2 H C N N O 3 NH H C 3 N A Fl B O redH 2
Isoalloxazine ring system NH 2 O AH2 O H O OH N P N P H O O 2 C CH N 2 N HO H O H H HO H FMN H H HO H OH H H H H C N N O 3 FAD NH H C 3 N O
FIGURE 10.3 Riboflavin, FMN, and FAD. When associated with flavoproteins, the reductive and oxidative half-reactions for FMN
and FAD occur wherein the nitrogens at positions 1 and 5 are involved. Redox of the isoalloxazine ring involves the following tran-
sition: -N1H-C=C-N5H- ↔ -N1=C-C=N5-.
Transport to tissues can be perturbed by boric acid
In humans, signs of riboflavin deficiency include
and certain drugs, e.g., theophylline and penicillin, which
lesions of the oral cavity, the lips, and the angle of the
displace riboflavin (or FMN) from known binding pro-
mouth (cheilosis), inflammation of the tongue (glossitis)
teins (e.g., albumin > globulins > fibrinogen).
and accompanying seborrhic dermatitis. Like other
Once in cells, riboflavin is phosphorylated to FMN. In
severe B-vitamin deficiencies, the filiform papillae of the
most cells about 20% of the riboflavin is present as FMN
tongue may also be lost with changes in color from pink
and of the remainder, over 70% is present as FAD with 1
to magenta. Anemia and increased vascularization of the
or 2% as free riboflavin. Urine is the major route of excre-
eye are present in some animals with riboflavin defi-
tion for riboflavin, although some FMN is excreted in bile ciency. (10 and refs. cited therein).
4. Food Sources and Stability
3. Metabolism and Requirements
The best sources of riboflavin are milk and dairy prod-
The requirements for riboflavin, 1.1 to 1.3 mg/day, are
ucts. Other good sources are meats, especially liver, eggs,
lower than those for niacin or ascorbic acid because
dark green leafy vegetables, and fortified breakfast cere-
riboflavin is tightly associated with the enzymes that
als (4). Today, milk and breakfast cereals make the great-
serve as a cofactor, and accordingly turns over much
est contributions to riboflavin intake in the U.S. and the
slower than niacin or ascorbic acid (Table 10.1; 4). In
U.K. Riboflavin is stable to acid pH, heat, and oxygen,
some cases, FMN is covalently bound (e.g., as in succinic
but is very susceptible to destruction by light, resulting in
dehydrogenase). Riboflavin is not toxic, primarily
the inactive compounds lumiflavin and lumichrome
because of its regulated uptake at the intestinal level, and
(Table 10.2; 13). These compounds and other oxidative
its relatively low solubility compared to other B-vitamins.
products can contribute to off-flavors due to oxidative The Water-Soluble Vitamins 10-9
damage to milk lipids. Milk exposed to visible spectrum
pathway is ribose-5-phosphate, a phosphorylated 5-carbon
light will lose 50% of its riboflavin content after two
sugar, required for the synthesis of the high-energy ribonu-
hours, thus the importance of packaging milk in opaque
cleotides, ATP, GTP, the nucleic acids, DNA and RNA, and containers.
the niacin-containing coenzyme NADPH, which is essential
for a number of biosynthetic reactions. A deficiency of thi-
IV. B-VITAMINS IMPORTANT TO SPECIFIC
amin can lead to decreased production of NADPH, which
impacts synthetic processes, such as fatty acid biosynthesis.
FEATURES OF CARBOHYDRATE,
In addition, it is evident from the neurological disorders
PROTEIN, OR LIPID METABOLISM
caused by thiamine deficiency that thiamin plays a vital role A. T
in nerve function. In the brain, TTP is proposed to function HIAMIN
in ion transport. The concentration of thiamin in the brain is
Studies on thiamin played an important role in the devel-
resistant to changes in dietary concentration. Stimulation of
opment of early concepts on the function and importance
nerves results in the release of thiamine monophosphate and
of vitamins. For example, the demonstration by the Dutch
free thiamine with accompanying decrease of cellular thi-
medical officer, Christian Eijkman, that polyneuritis could
amine pyrophosphate and thiamine triphosphate and
be produced in an experiment animal by dietary manipu-
changes in Na+ and K+ gradients (19).
lation was conceptually very important. Eijkman and his
Human thiamin deficiency, beriberi, is termed dry,
colleagues fed a diet of polished rice, presumably low in
wet, or cerebral, depending on the systems that involved.
thiamin, to chickens (one of the first uses of an animal
The main feature of dry (paralytic or nervous) beriberi is
model to study disease) and observed characteristic fea-
peripheral neuropathy, including abnormal (exaggerated)
tures of beriberi, such as head retraction, a common neu-
reflexes, diminished sensation, and weakness in the legs
rological sign in animals. The focus on rice and the
and arms. Muscle pain and tenderness are also features.
observation that there appeared to be a curative principle
When cardiovascular manifestations occur, the term wet
in rice bran led to the eventual isolation of thiamin. This
beriberi is used. Signs include a rapid heart rate, cardiac
sequence of events and experimental protocols provided
enlargement, and edema. Ultimately, congestive heart
the underpinnings that led to the discovery of vitamins as
failure may be the cause of death. Cerebral beriberi can precursors to cofactors (19).
lead to Wernicke’s encephalopathy and Korsakoff’s psy-
chosis. Signs include abnormal eye movements, stance 1. Chemistry
and gait abnormalities, and abnormalities in mental func- tion, e.g., confusion (19).
The five-member (thiazole) ring of thiamin contains an
arrangement of atoms (-N=CH-S-) called an ylid. The
3. Requirements, Pharmacology, and Toxicity
central carbon has carbanion character that acts as an elec-
tron-rich center for reactions that are commonly charac-
The requirement for thiamin is 1.1 to 1.2 mg per day or 0.5
terized as decarboxylations and transketolations (Figure
mg per 1000 kcal or 4 MJ of diet (4; Table 10.1). Factors
10.4). Thiamin is not stable to heat or alkali.
that influence requirements are inadequate intake, strenu-
ous physical exertion, fever, pregnancy, breastfeeding, and
2. Absorption, Tissue Distribution, and
adolescent growth, or exposure to antagonists, such as tan- Metabolism
nins, alcohol (sufficient to cause inflammatory bowel con-
ditions), and thiaminases, enzymes in many foods,
Thiamin occurs in the human body as free thiamin and its
particular mollusks, and the muscle of fish. Individuals
mono-, di-, and triphosphorylated forms: thiamin
who habitually eat raw freshwater fish, raw shellfish, and
monophosphate, thiamin pyrophosphate (TPP), which is
ferns are at higher risk of thiamin deficiency because these
designated as thiamin diphosphate, and thiamin triphos-
foods contain a thiaminase. For example, an acute neuro-
phate (TTP), which is found mostly in neural tissue. TPP
logical syndrome (seasonal ataxia) in Nigeria has even
and TTP are the cofactor forms of thiamine.
been associated with thiamin deficiency precipitated by a
Thiamin is essential to the utilization of carbohydrates
thiaminase in African silkworms, a traditional high-protein
as energy sources, because of roles in TCA cycle regulation, food for some Nigerians.
and perturbations in the pentose phosphate – related carbo-
Antagonists include pyrithiamine and oxythiamine,
hydrates pathways. In the TCA cycle, the principle enzymes
which act to inhibit the phosphorylation of thiamin.
are pyruvate dehydrogenase and α-ketoglutarate dehydroge-
Amprolium (coccidiostat) inhibits thiamin absorption.
nase. These enzymes catalyze the decarboxylations of pyru-
vate and α-ketoglutarate. TPP is also a cofactor for
4. Food Sources and Stability
transketolase reactions. Transketolation is a central activity
in the metabolic sequence known as the pentose phosphate
Thiamin is present in most animal and plant foods, but is
pathway. One of the most important intermediates of this
especially prominent in whole grains, organ meats, pork, 10-10
Handbook of Food Science, Technology, and Engineering, Volume 1 NH2 CH3 (A) OH N N+ N − C S Thaimin H C 3 H (B) O O N+ N+ N − O O − C -S H+ C −S C S − O R H HO R + HO R CO 2 Ketoacid Resonance stabilized
Transfer of the decarboxylated product to coenzyme A
AcetyI-S-CoA (from pyruvate) N+ C −S
Succinyl-S-CoA (from a-ketoglutarate) (−) HO R CH2OH (C) CH O 2 OH CHO O H OH Transketolase CHO HO H + HO H H OH H OH + H OH O O H OH O H OH H C P OH 2 P OH H C 2 H C O 2 P OH OH OH OH H2C P OH OH
FIGURE 10.4 Thiamin. The carbanion character of thiamin [A] facilitates the non-oxidative and oxidative decarboxylation of
α-ketoacids [B] and transketolase reactions [C].
eggs, nuts, and legumes. Many food cooking and processing
in a number of other enzymes where thioesters facilitated
conditions will negatively affect thiamin content, as it is
transfer reactions are essential (5).
unstable in neutral and alkaline conditions, and with heat,
oxygen, and sulfur exposure (Table 10.2). Milling of whole
1. Absorption, Tissue Distribution, and
grains will remove the bran where thiamin and other B vita- Metabolism
mins are in greatest concentrations; however, the current
practice of fortification has restored thiamin levels in flours
Both Coenzyme A (CoASH) and ACP are present in and grain-based products (13).
foods. Consequently, absorbed pantothenic acid must first
be released from CoASH and ACP, steps that involve the
actions of peptidases and nucleosidases. The mechanism B. PANTOTHENIC ACID
of absorption of pantothenic acid in the small intestine
Roger William discovered pantothenic acid in 1933, and
involves the same carrier-mediated Na-dependent sys-
observed that it was an essential growth factor for yeast
tem that transports biotin (15). Similarly, colonic absorp-
and lactic acid bacteria. Over the next 15 years, pan-
tion of pantothenic acid was found to involve the
tothenic acid was shown to be essential in animals.
biotin-Na-dependent, carrier-mediated system. The
Pantothenic acid serves as a component of coenzyme A
interaction between biotin and pantothenic acid transport
(Figure 10.5) and as a part of phosphopantotheine, e.g., at
has also been described in other tissues such as the blood
the acyl carrier site in acyl carrier protein (ACP) (18).
brain barrier, the heart, and the placenta, but the impor-
Recently, pantothenic acid has been shown to be present
tance of this interaction is not understood. The Water-Soluble Vitamins 10-11
2. Metabolic Functions and Requirements C. PYRIDOXINE
CoASH is the principle moiety for the vectoral transport
Pyridoxine or vitamin B-6 is a collective term for pyri-
of acyl and acetyl groups in synthetic and catabolic reac-
doxine, pyridoxal, and pyridoxyl amine. Pyridoxine is
tions. A deficiency is characterized by impaired acetyl and
most abundant in plants; pyridoxal and pyridoxyl amine
acyl metabolism. The ability to utilize fatty acids as fuels
are most abundant in animal tissues. The active form of
is clearly compromised in experimental pantothenic acid
vitamin B-6 is phosphorylated at the 5 position, e.g., pyri-
deficiency. There is also an increased production of short doxal-5-phosphate (7).
chain fatty acids and ketone bodies, which can lead to
severe metabolic acidosis. Dermal lesions occur because 1. Chemistry
of impaired fatty acid metabolism. Selected aspects of
pantothenic acid utilization and CoASH regulation are
The most common of the reactions catalyzed by vitamin given in Figure 10.5.
B-6 containing enzymes is the transaminase reaction.
The requirement for pantothenic acid is about 5 mg
Transaminations are essential to the interconverson of
per day or 2 mg per 1000 kcal (~4 MJ) of diet. Even at
amino acids to corresponding α-keto acids. The mecha-
gram intakes, pantothenic acid does not appear to be
nism is also useful for reactions important to producing toxic (4).
racemic amino acid mixtures (e.g., the conversion of
L-alanine to D-alanine) and α, β−addition or elimination α, β−
3. Food Sources and Stability reactions. An example of an elimination reaction is
the conversion of serine to pyruvic acid or the conversion
Good dietary sources of pantothenic acid are meats, organ
of homocysteine plus serine to cystathionine. The basic
meats, eggs, nuts and legumes, cereals and some vegeta-
feature of the transamination mechanism is electron with-
bles such as broccoli. The vitamin is stable in neutral pH,
drawal from the α-carbon resulting in a proton liberation,
but unstable outside of this range, and also susceptible to
which sets the stage for subsequent substitution and addi-
degradation by heat treatment.
tional reactions (Figure 10.6; 7). Pantothenic acid ATP Pantothenic acid
Inhibited by CoA and CoA derivatives kinase ADP CoA inhibition is reversed by carnitine 4 -phosphopantothenic acid
4 - phosphopantothenoylcysteine CTP + Cysteine synthetase CDP + Pi 4 -phosphopantothenoylcysteine
4 -phosphopantothenoylcysteine decarboxylase CO2 4 -phosphopantetheine Dephopho-CoA pyrophosphorylase ATP 3 ,5 -ADP AMP + PPi CoA hydrolase 4 -dephospho-coenzyme A Dephospho-CoA kinase ATP ADP Coenzyme A
FIGURE 10.5 Synthesis of Coenzyme A (CoASH) from pantothenic acid. The synthesis of CoASH from pantothenic acid is regu-
lated in part by feedback inhibition, e.g., by CoA derivatives at the step catalyzed by pantothenic acid kinase. Carnitine also plays a role in regulation. 10-12
Handbook of Food Science, Technology, and Engineering, Volume 1
The second most common reaction involves electron
Another important function of vitamin B-6 (as pyri-
withdrawal from the α-carbon and carboxylic acid group
doxal-5-phosphate), independent of amino acid metabo-
carbon, which results in decarboxylation (Figure 10.6).
lism, is its role in glycogen phosphorylase. Glycogen
Decarboxylation reactions include the conversions of tyro-
phosphorylase catalyzes the hydrolysis of ether (α-1 to 4
sine to tyramine, 5-hydroxytryptophan to serotonin, histi-
C-O-C) bonds in glycogen to form glucose-6-phosphate.
dine to histamine, and glutamate to γ−aminobutyric acid
Ether bonds are best catalyzed through acid-mediated
(GABA). Signs of convulsions associated with vitamin
mechanisms. The acid proton in this instance is derived
B-6 deficiency are attributed to insufficient activity of
from the phosphate group of pyridoxal-5-phosphate. This
pyridoxal-5-phosphate-dependent, L-glutamate decar-
important mechanism was only elucidated recently. boxylase.
Previously, it was speculated that the association of vita-
A third type of reaction involves electron withdrawal
min B-6 with glycogen phosphorylase was primarily from the β, -
γ carbons of amino acids (Figure 10.6). This sets
some type of storage mechanism for B-6.
the stage for hydride condensations or aldol reactions. A
good example of an aldol reaction is the conversion of ser-
2. Absorption, Tissue Distribution, and
ine to glycine with the transfer of the β-carbon (as formalde- Metabolism
hyde) to another vitamin cofactor, tetrahydrofolic acid in
single carbon reactions (see the next section). Another
Vitamin B-6 is absorbed in the upper gut by energy-
example of a hydride condensation is the formation of
dependent pathways. Muscle, kidney, and liver are rich in
α-aminolevulinic acid, the first step in heme biosynthesis.
pyridoxal enzymes, e.g., glycogen phosphorylase (in Enzyme Enzyme (CH HB 3)4 (CH3)4 NH2 H O HN+ H C C 3− H+ H+ −O CH 3− 2OPO3 −O CH2OPO3 H + H + 3C H H N H+ H+ 3C N H H H H : O H Enzyme H C COOH (CH3)4 : NH2 NH2 Transamination
or , elimination/addition reactions H
R-group eliminations or additions H C COOH NH+ Decarboxylation HC 3− −O CH2OPO3 H + 3C H N H
FIGURE 10.6 Pyridoxal-5-phosphate. Vitamin B-6, as pyridoxal-5-phosphate, is linked to pyridoxal-5-phosphate containing
enzymes by an dissociable imine linkage with a lysyl residue at the enzyme’s active site. By a process of transamination given amino
acids are transferred to pyridoxal-5-phosphate. Depending on the enzyme and nature of the enzymatic process, transamination reac-
tions, α,β-elimination/addition, R-group elimination/addition, or decarboxylation reactions can occur. The Water-Soluble Vitamins 10-13
muscle) and amino acid catabolizing enzymes and
poisoning, which was associated with poor growth, alope-
transaminases (in liver and kidney).
cia, and dermatitis in animals exposed to raw eggs and egg
The major form of vitamin B-6 in cells is pyridoxal
whites as a primary source of dietary protein. It was even-
–5-phosphate. The conversion of the pyridoxal form of
tually found that biotin-binding factors in eggs could
the vitamin to pyridoxic acid signals elimination from the
reduce biotin availability (see below). body (7 and refs. cited).
3. Requirements, Pharmacology, and Toxicity 1. Chemistry
Vitamin B-6 deficiency is rarely seen as most diets provide
Biotin functions in enzymatic carboxylation reactions as a
adequate amounts. Normally, the vitamin B-6 is met at 1.3
cofactor for CO – fixing enzymes. Examples include: 2
or more mg per day or about 0.3 mg per 1000 kcal (4MJ)
acetyl CoA carboxylase, which is essential for fatty acid
of diet (Table 10.1; 4). Drug-induced vitamin B-6 defi-
synthesis; propionyl CoA carboxylase, which participates
ciency can occur following administration of the tubercu-
in odd chain fatty acid metabolism; pyruvate carboxylase,
lostatic drug, isoniazid (isonicotinic acid hydrazide). This
which is involved in the formation of oxaloacetate, an
drug forms hydrazone derivatives with pyridoxal or pyri-
important obligatory step in reverse glycolysis and gluco-
doxal phosphate, which can then act to inhibit pyridoxal-
neogenesis; and β-methylcrotonyl CoA, important in
containing enzymes. Patients receiving long-term isoniazid leucine metabolism (9).
therapy respond to the administration of supplemental vita-
Biotin is covalently bound in carboxylases and trans-
min B-6. Penicillamine (β-dimethylcysteine) used in the
carboxylases by peptidyl linkage between the carboxylic
treatment of Wilson’s disease also can interfere with nor-
acid moiety of biotin and the ε-amine function of peptidyl
mal B-6 metabolism due to the formation of thiazole deriv-
lysine (extends from the side chain associated with the
atives. A naturally occurring antagonist to vitamin B-6,
sulfur-containing ring of biotin, which is only partially
linatine (1-amino-D-proline) is present in flax seed, which
depicted in Figure 10.7). The biotin-lysine adduct is
forms a stable product with pyridoxal phosphate (7).
called biocytin. Biocytin can be cleaved by the enzyme
The most important signs of B-6 deficiency relate to
biocytinase to generate free biotin.
defects in amino acid metabolism. Neurologic signs occur
because of reduced synthesis of important biogenic amines
2. Metabolism and Requirements
from amino acid precursors (7), and anemia is a conse-
quence of decreased heme synthesis. Adverse effects have
Biotin is found in highest concentrations in the liver. In
only been documented from vitamin B-6 supplements and
plant foods, biotin is present in relative high concentrations
never from food sources. Chronically high doses of pyri-
in most cereals. Dietary biotin exists in free and protein
doxine can result in painful neurological symptoms known
bound forms. The latter form is digested first by gastroin-
as sensory neuropathy. Symptoms include pain and numb-
testinal proteases and peptidases to generate biocytin
ness of the extremities, and in severe cases difficulty walk-
(N-biotinyl-L-lysine) and eventually free biotin plus lysine.
ing. Sensory neuropathy typically develops at doses of
Like the other water-soluble vitamins, biotin is taken up by
pyridoxine in excess of 1,000 mg per day. At intakes below
specialized Na-dependent carrier-mediated mechanisms.
200 mg/day, sensory neuropathy is not observed.
At the basolateral membrane, biotin exits cells via a Na-
dependent carrier system. In adult humans, biotin uptake is
4. Food Sources and Stability
significantly higher in the duodenum and jejunum com-
The richest sources of vitamin B-6 are meats and whole
pared to ileum. As noted previously, biotin shares the trans-
grains. Heat and light are the two primary treatments that
porter with pantothenic acid (15, 18).
negatively affect the stability of the vitamin (Table 10.2).
When biotin-containing carboxylases are degraded in
Similar to other B vitamins, milling of whole grains and
cells, biotin is also released as biocytin. Cellular biocyti-
removal of the bran will result in significant losses of
nases catalyze its cleavage to release free biotin for reuti-
pyridoxine. Fortification of cereal products will restore B
lization. In the absence of this enzyme, biotin reutilization vitamin levels (4).
is compromised. Re-biotinylation requires ATP to catalyze
the formation of a peptide bond between biotin and a lysyl
group at the active center of targeted carboxylases (9).
V. B-VITAMINS INVOLVED IN SINGLE
The nutrition need for biotin is expressed as an CARBON AND CO TRANSFER 2
“Adequate Intake” and amounts to ~25 micrograms per REACTIONS
day (Table 10.1; 4). Reasons for the relatively lower A. B
requirements are: biotin is covalently attached to a limited IOTIN
number of enzymes that it serves as a cofactor; biotin is
Biotin was discovered following a long search for factors
extensively reutilized; significant amounts of biotin are
important to the understanding of the condition, egg white
produced by the gut microflora. 10-14
Handbook of Food Science, Technology, and Engineering, Volume 1 X X O O O O 2′ HN3′ 1′ N−H HN N HN N− CO2 C O OPO3H S S S Product - CO2 Substrate FIGURE 10.7 Biotin.
Nevertheless, nutritional problems associated with
1940s and 1950s and careful clinical and basic studies even-
biotin status can occur. Biotin and biocytin have a high
tually led to the isolation of folic acid and vitamin B-12.
affinity for certain proteins, particularly avidin in egg
white. The consumption of raw egg albumin can induce 1. Chemistry Folic Acid
biotin deficiency, due to the strong association of biotin
with avidin rendering dietary biotin unavailable. Ingestion
The folates are a group of heterocyclic compounds com-
of significant quantities of raw egg white by fur-bearing
posed of 4-(pteridin-6-ylmethyl)-aminobenzoic acid con-
animals and pigs has led to the condition initially
jugated with one or more L-glutamate units. Folate and
described as “egg white injury.”
folic acid are the preferred synonyms for pteroylglutamate
Biotin deficiency leads to impairment of gluconeoge-
and pteroylglutamic acid, respectively, and designate any
nesis and fat metabolism. Biotin deficiencies can also
members of the family of pteroylglutamates having vari-
induce severe metabolic acidosis. The inability to carry
ous levels of reduction of the pteridine ring, one-carbon
out fat metabolism, also markedly affects the dermis.
substitutions, and differing numbers of glutamate residues
Alopecia and dermatitis are characteristics of biotin defi- (Figure 10.8; 2). ciency in most animals (9).
The reactions that involve folic acid include the gen-
eration and utilization of formaldehyde, formimino, and
methyl groups. For these conversions to occur, folic acid
3. Food Sources and Stability
must be reduced in the form of tetrahydrofolic acid
Biotin in animal foods is found in highest concentrations
(THFA). Reduction brings the nitrogen at positions 5 and
in the liver, but also in meats, milk, and egg yolk. In plant
10 closer together and changes their electrochemical
food, biotin is present in relative high concentrations in
properties, which facilitates the formation of the various
most cereal grains, as well as nuts, mushrooms, and some
THFA single carbon derivatives.
green vegetables. It is relatively stable to most treatments,
The formyl, methanyl, and methylene forms of THFA
with the exception of heat and alkaline conditions, which
are utilized for purine synthesis and in thymidylate (i.e., result in minor losses.
DNA-related) synthesis. These reactions are essential to
cell division and proliferation. Folate also participates in
B. FOLIC ACID AND VITAMIN B-12
reactions involved in the interconversion and catabolism
of amino acids. Much of the folate in the body is also in
Vitamin B-12 and folic acid will be discussed together,
the form of methyl-THFA for eventual methyl transfer
because their functions and metabolism are uniquely
reactions. Removal of the methyl group from methyl-
linked (Figure 10.8). Knowledge regarding folic acid and
THFA and its eventual irreversible transfer to methionine
B-12 evolved from efforts to better understand macrocytic
by the vitamin B-12 requiring enzyme, methionine syn-
anemias and certain degenerative neurologic disorders
thetase, represents an important interaction between folic
(8). The Scottish physician, Combe, recognized in the acid and vitamin B-12 (1, 2).
early 1800s that certain forms of macrocytic anemia
appeared related to a disorder of the digestive organs. In
2. Chemistry: Vitamin B-12
classic studies by Minot, Murphy, Castle, and others, it
became clearer that the disorder was associated with gas-
Vitamin B-12 consists of a porphyrin-like structure of
tric secretions and in some cases could be reversed by
tetrapyrrole rings with a monovalent cobalt metal at the
consuming raw or lightly cooked liver.
center (Figure 10.9; 1). A 5,6-dimethylbenzimidazolyl
In parallel studies, folic acid was also associated with
nucleotide is also linked to the tetrapyrrole rings via a
macrocytic anemia. Large-scale efforts throughout the
phosphate sugar linkage. Vitamin B-12 catalyzes the The Water-Soluble Vitamins 10-15 H N N H N 2 1 8 2 7 3 6 O CO O CO N 9 2 2 4 5 N CH2 H H H HN C N CH (CH C 2)2 N CH (CH2)2 CO2 OH 10 n R AdoMet PPi + Pi R − CH3 ATP Methionine Methylations AdoHcy H o 2 Ser B-12 THFA Ado H o 2 ∗ Homocysteine Gly Ado ∗ 5 ∗ N , N10-CH -THFA N5-CH -THFA 2 3 dUMP cystathionine DNA synthesis dTMP N5, N10-CH=−THFA Hyperhomocysteinemia N10-CHO −THFA CO Purine syntheis 2 COO−
FIGURE 10.8 Folic acid. Basic features of the folic acid structure are shown. Folate is essential to: 1) methylation reactions (via
the vitamin B-12-dependent methionine synthetase step), 2) DNA synthesis (via single carbon transfer from N5, N10-methylene-THFA in
the conversion deoxyuridylate to deoxythymidylate), 3) purine synthesis (via transfer of a single carbon from N10-formyl-THFA into the
purine pathway). Not shown are reduction steps that result in the regeneration of THFA (at positions 5 and 8) from oxidized folic acid.
conversion of homocysteine to methionine via transfer of
dietary folate. A specialized carrier-mediated system is
a methyl moiety derived from methyl-THFA. Vitamin B-
involved in folate uptake across the apical membrane of
12 in the form of the adenosyl derivative can also catalyze
the absorptive cells, as well as other cells. The factors that
the conversion of methylmalonyl CoA to succinyl CoA.
negatively affect intestinal folate absorption are alcohol
Deficiency of vitamin B-12 leads to intracellular accumu-
consumption and the use of certain pharmacological
lation of metabolites of these two reactions, homocysteine
agents like sulfasalazine and phenytoin (Dilantin). An
and methylmalonic acid (1, 2, 8, 13, 16).
important step in the digestive process is the conversion of
polyglutamyl folate to monoglutamyl folate by intestinal polyglutamyl hydrolase.
3. Digestion, Metabolism, and Tissue Distribution
There are a number of substances in unprocessed
Folate absorption requires processing of food-derived
foods (e.g., uncooked beans) that act as folate hydrolase
folates from poly- to monoglutamyl forms. The proximal
inhibitors. In plasma, there are specific carrier proteins,
part of the small intestine is the main site of absorption of
which take folic acid to targeted cells. In this regard, 10-16
Handbook of Food Science, Technology, and Engineering, Volume 1 Cyanocobalamin O O NH C 2 H H C CH 2C 2 3 CH3 CH2 C NH2 H2 N C C H2 CH2 CH2 C NH2 O H C 3 CN N N O H3C Co H O N N CH3 H N C CH 2 2 CH3 O H CO CH CH 2 2 CH CH CH NH NH 3 CH 2 3 2 C 2 2 N N CH N 2 H C H N 3C N CH N 3 O O − H O 2 P Ribose O N CH3 H O 2 O OH ATP CH2 CH3 N5-CH -THFA CH2 3 −CH2 CH CH 2 2 O C H H3C N N 2 HO CH2 CH2 H3C CH Co 3 N N H H3C N N Co CH H 3 −CH2 N N CH CH 3 3 − H C CH2 CH2 CH CH 2 3CH 2 CH 3 3 CH C CH − H C C C C 2 2 CH CH 2 3 3 X H X Ado CH Ado CH (Substrate) 2 3 C C N N N N C C X H Co(II) Co(II) X N N N N Ado CH3 Ado CH2 N N N N C C C C Co(II) Co(III) H X X N N N N Ado CH Ado CH 2 3 N N N N C C Co(II) Co(II) H X N N N N (Product) COCoA COCoA Vitamin B-12 H3C C COOH H COOH H 2C C H2 Methylmalonyl CoA Succinyl CoA
FIGURE 10.9 Cyanocobalamin. Commercial preparations of vitamin B-12 usually have a cyano group coordinated with the cobalt
(associated with the corrin ring of vitamin B-12). In an aqueous environment the cyano group can be displaced by water for the even-
tual transfer of a methyl group (donated by N5-methyl-THFA) or an adenosyl moiety (from ATP). Methylated vitamin B-12 serves as
a cofactor for methionine synthetase (see Figure 10.7). Adenosylated vitamin B-12 serves as a cofactor for unusual isomerase reac-
tions, such as the conversation of methylmalonyl CoA to succinyl CoA. A mechanism for this process is shown, which involves the
redox of cobalt in the corrin ring of cobalamin (Co+3«Co+2). The Water-Soluble Vitamins 10-17
ethanol can also markedly decrease folate biliary secretion,
the etiology of coronary artery disease because of the
thereby reducing availability to body tissues from liver. In
association of elevated homocysteine as a risk factor in
tissues, the distribution of folates is largely dependent on vascular disease (8).
the concentration of folate dependent enzymes. Methyl-
The movement of single carbon units from folate
THFA mono glutamate is rapidly transported (bound to
through methionine synthetase in the formation of
carrier proteins) to liver. Uptake proceeds through a
methionine is also important for S-adenosylmethionine
receptor-mediated endocytotic process and is then quickly
(SAM) formation. SAM is essential to methylation of
converted to folate in various polyglutamyl forms (2).
phospholipids, and production of methylated forms of
Steps important to processing of vitamin B-12 involve
various amino acids and carbohydrates. SAM also serves
first release from foods under acidic conditions. Vitamin
as the methyl source in DNA methylation.
B-12 then binds to proteins produced by cells of the gas-
Deficiencies of both vitamin B-12 and folic acid pro-
tric fundus, pancreas, and salivary gland. Two proteins
duce clinical signs of macrocytic anemia and disyn-
have been identified, which are designated as R protein
chronies in growth and development owing to the
and intrinsic factor. Vitamin B-12 first binds to R protein
importance of folic acid to purine and DNA synthesis.
and is released in the intestinal lumen by the action of
Chronic deficiencies of either folic acid or B-12 can also
pancreatic and intestinal proteinases and peptidases, to
promote fatty liver disease and indirectly influence extra-
allow vitamin B-12 to bind to intrinsic factor. Intrinsic
cellular matrix maturation stability by causing abnormal
factor is a small-molecular-weight glycoprotein, which is
elevations in homocysteine (1, 2, 8, 11, 13, 16). Such
made in stomach parietal cells (1). The vitamin B-12 –
signs and symptoms are attributable to both THFA and
intrinsic factor complex then interacts with receptors on
B-12 deficiencies, because of the integral relationship of
the intestinal brush border localized in the midgut (i.e.,
vitamin B-12 to THFA regeneration. Dietary intakes of
ileum). Interference with R protein or intrinsic factor pro-
folic acid, sufficient to maintain functional THFA levels,
duction, or inflammatory disease affecting the ileum, or
can mask the initial signs of vitamin B-12 deficiency (e.g.,
overproduction of intestinal microflora can adversely
macrocytic and megaloblastic anemia). Prolonged vitamin
affect the availability of vitamin B-12 (1).
B-12 deficiency in humans results in serious neurologic
After vitamin B-12 is taken up by luminal cells, it is
disorders due to degeneration of the myelin sheath (1).
transported into the lysosomes where the vitamin B-12 –
The requirement for folic acid is 400 μg per day
intrinsic factor complex is degraded and the vitamin
(Table 10.1; 4). However, there are some conditions in
B-12 is released and vectorally directed for release into
which the folic acid requirements are conditionally high,
plasma. Vitamin B-12 is transported in plasma by one of
e.g., when either natural or pharmacological folic acid
three specific transport proteins, transcobalmin I, II, or III.
agonists are present in the diet. A range of genetic poly-
Transcobalmin I carries vitamin B-12 to the liver.
morphisms in proteins important to folate metabolism can
Transcobalmin II carries vitamin B-12 from the liver to
also influence the folic acid requirement. Alterations in
peripheral tissues. Note that the processing of folate and
folate hydrolase, folate reductase, or methionine syn-
vitamin B-12 in and out of tissues differs from that for
thetase that cause changes in the affinity for folate bind-
other water-soluble vitamins. The process is receptor
ing are all known to impact folate requirements (2).
mediated and endocytotic, in contrast to involvement of
The requirement for vitamin B-12 is 2.4 μg per day or
an active sodium-dependent transporter (1).
1 μg per 1000 kcal (~4 MJ) of diet (Table 10.1; 4).
Although vitamin B-12 deficiency is rare, disease of the
4. Requirements, Pharmacology, and Toxicity
stomach, proximal duodenum, or ileum and pancreatic
insufficiency can affect folic acid and vitamin B-12
Decreased production of methanyl and methylene forms absorption, respectively.
of folate can result in decreased purine synthesis and
thymidylate formation from uridylate. The latter can cause
5. Food Sources and Stability
replicating cells to arrest in the S-phase of the cell cycle.
Epithelial cells are often most affected by folate deficiency
Folic acid: Foods that are particularly high in folate are
(2, 8). The consequences range from megaloblastic ane-
oranges, green leafy vegetables, and whole grains.
mia to growth retardation. Accordingly, aggressive supple-
Fortification with folic acid to all enriched cereal grain
mentation of folic acid has been associated with a decrease
foods was begun in the U.S. in 1998 by mandate of the
in the appearance of certain developmental defects, e.g.,
Food and Drug Administration (11). The primary objec-
abnormal neutral tube closure (2, 11, 13, 16).
tive of this program was to provide increased folic acid to
As noted, the most important link between folate and
women of childbearing age in order to decrease the occur-
vitamin B-12 occurs at the methionine synthetase step,
rence of neural tube defects (NTD). The fortification pro-
where 5-methyl THFA serves as a substrate with homo-
gram has successfully decreased NTDs by 19%, but any
cysteine to form methionine. In this regard, recent studies
longer term health benefits on other diseases is unknown
have suggested that low folate levels may play a role in
at this time. Folic acid is stable in acid pH, but is rapidly 10-18
Handbook of Food Science, Technology, and Engineering, Volume 1
destroyed in neutral or alkaline conditions and is unstable
2. Brody T & Shane B, Folic Acid In: Handbook of
to prolonged heat, oxygen, and light exposure.
Vitamins, 3rd Edition, Eds: Rucker, RB, Suttie, JW,
Dietary sources of B-12 are meats with liver being the
McCormick, D, & Machlin, LJ, Dekker Inc., New York,
highest followed by dairy products and some seafoods pp. 427–462, 2001.
(1, 2, 4). B-12 is also present in yeast and fortified cere-
3. Carr AC & Frei B, Toward a new recommended dietary
als. The reliance on primarily animal products for vitamin
allowance for vitamin C based on antioxidant & health
effects in humans. Am J Clin Nutr 69:1086–1107,
B-12 means that strict vegetarians are potentially at risk 1999.
for B-12 deficiency. B-12 is relatively stable and
4. Food & Nutrition Board, Institute of Medicine. Dietary
destroyed only by extended heat treatments and exposure
reference intakes. Washington, DC: National Academy
to light and oxygen (Table 10.2). Press, Washington, D.C., 1998.
5. Joshi AK, Zhang L, Rangan VS, & Smith S, Cloning,
expression, and characterization of a human 4-phos- VI. CONCLUDING COMMENTS
phopantetheinyl transferase with broad substrate speci-
Additional compounds, mostly derived from carbohydrate
ficity. J Biol Chem. 278:33142, 2003.
and amino acid metabolites, are listed with the vitamins,
6. Kirk, JB & Rawling JM. Niacin, In: Handbook of
Vitamins, 3rd Edition, Eds: Rucker, RB, Suttie, JW,
because of conditional dietary needs or functions in meta-
McCormick, D, & Machlin, LJ, Dekker Inc., New York,
bolic regulation. Many of these compounds typically per- pp. 213–254, 2001.
form specialized transport function, particularly in relation
7. Leklem JE, Vitamin B-6 In: Handbook of Vitamins, 3rd
to fatty acids. Examples include choline (a major source of
Edition, Eds: Rucker, RB, Suttie, JW, McCormick, D, &
methyl groups in the diet), inositol (important in signal
Machlin, LJ, Dekker Inc., New York, pp. 339–396, 2001.
transduction), and carnitine (required for the transportation
8. Mason JB, Biomarkers of nutrient exposure &
of fatty acids from the cytosol into the mitochondria).
status in one-carbon (methyl) metabolism. J Nutr
Taurine, queuine, the ubiquinones (coenzyme Q), the 133:941S–947S, 2003.
tetrahydrobiopterins, Pyrroloquinoline quinone (17), and
9. Mock DM, Biotin In: Handbook of Vitamins, 3rd Edi-
lipoic acid could also be added to the list, because of their
tion, Eds: Rucker, RB, Suttie, JW, McCormick, D, &
Machlin, LJ, Dekker Inc., New York, pp. 397–426, novel roles in metabolism. 2001.
Regarding the need for vitamins, acute deficiencies for
10. Powers HJ, Riboflavin (vitamin B-2) and health, Am J
most water-soluble vitamins can be induced under experi-
Clin Nutr 77:1352–1360, 2003.
mental conditions. Frank deficiency diseases are rarely seen
11. Quinlivan EP & Gregory JF, Effect of food fortification
in most populations unless specific food sources are severely
on folic acid intake in the United States. Am J Clin Nutr
limited due to food distribution problems, environmental 77:221–225, 2003.
problems such as drought, or severe economic constraints
12. Johnston CS, Steinberg FM, & Rucker RB, Ascorbic
that limit access to food. However, marginal deficiencies can
acid, In: Handbook of Vitamins, 3rd Edition, Eds:
be observed when individuals consume a monotonous diet
Rucker, RB, Suttie, JW, McCormick, D, & Machlin, LJ,
in which relatively few foods constitute a source of calories.
Dekker Inc., New York, pp. 529–554, 2001.
Genetic polymorphisms in proteins involved in vitamin
13. Reddy MB & Love M, The impact of food processing
on the nutritional quality of vitamins and minerals. Adv
metabolism may also affect vitamin nutriture and result in
Exp Med Biol. 459:99–106, 1999.
increased needs. When a defect is associated with a vitamin
14. Rucker RB & Steinberg FM, Vitamin requirements.
deficiency, what often defines why the defect occurs is the
Relationship to basal metabolic need & functions.
K for the association or binding of the vitamin with pro- m
Biochem Mol Biol Educ 30:86–89, 2002.
teins associated with the defect (e.g., a specific enzyme). In
15. Said, HM, Cellular uptake of biotin: mechanisms and
such cases, the K or related constants is usually high. In
regulation. J Nutr. 129:490S–493S, 1999. m
addition, disturbances of absorption (e.g., pancreatic insuffi-
16. Selhub J Folate, vitamin B12 & vitamin B6 & one car-
ciency, biliary obstructions, alcohol, enteropathies), antago-
bon metabolism. J Nutr Health Aging, 6:39–42,
nists (e.g., antibiotics, tannins, caffeic acid, alcohol), or 2002.
metabolic conditions (e.g., pregnancy, diabetes) may con-
17. Steinberg F, Stites TE, Anderson P, Storms S, Chan I,
tribute to the increased need for given vitamins (14).
Eghbali S, & Rucker RB, Pyrroloquinoline quinone
improves growth and reproductive performance in mice
fed chemically defined diets. Exp Biol Med 228:160– 166, 2003. REFERENCES
18. Tahiliani AG & Beinlich CJ, Pantothenic acid in health &
disease. Vitam Horm. 46:165–228, 1991.
1. Beck WS, Cobalamin (Vitamin B12) In: Handbook of
19. Tanphaichitr V, Thiamine In: Handbook of Vitamins,
Vitamins, 3rd Edition, Eds: Rucker, RB, Suttie, JW,
3rd Edition, Eds: Rucker, RB, Suttie, JW, McCormick,
McCormick, D, & Machlin, LJ, Dekker Inc., New York,
D, & Machlin, LJ, Dekker Inc., New York, pp. 275–316, pp. 463–512, 2001. 2001.