-
Thông tin
-
Quiz
Dysregulation of oxidative phosphorylation in human diseases | Bài tiểu luận học phần Writing BC2 | Trường Đại học Quốc tế, Đại học Quốc gia Thành phố Hồ Chí Minh
Oxidative phosphorylation (OXPHOS) is the final stage of cellular respiration, which is counted as the most abundant source of Adenosine Triphosphate (ATP) among the three processes of cellular respiration. These three processes are Glycolysis, the Krebs cycle, and Oxidative phosphorylation, respectively. The fundamental mechanism of oxidative phosphorylation is to synthesize ATP based on accumulated proton via the electron transport chain (ETC). Fumarate that is derived from this process can also enter the Krebs cycle. OXPHOS is regulated by cellular energy demand. Several factors tightly control the regulation of OXPHOS including the concentration of ATP and ADP, availability of oxygen, electron carriers (NADH and FADH2), and membrane potential. Tài liệu giúp bạn tham khảo, ôn tập và đạt kết quả cao. Mời bạn đón xem.
Writing BC2 1 tài liệu
Trường Đại học Quốc tế, Đại học Quốc gia Thành phố Hồ Chí Minh 696 tài liệu
Dysregulation of oxidative phosphorylation in human diseases | Bài tiểu luận học phần Writing BC2 | Trường Đại học Quốc tế, Đại học Quốc gia Thành phố Hồ Chí Minh
Oxidative phosphorylation (OXPHOS) is the final stage of cellular respiration, which is counted as the most abundant source of Adenosine Triphosphate (ATP) among the three processes of cellular respiration. These three processes are Glycolysis, the Krebs cycle, and Oxidative phosphorylation, respectively. The fundamental mechanism of oxidative phosphorylation is to synthesize ATP based on accumulated proton via the electron transport chain (ETC). Fumarate that is derived from this process can also enter the Krebs cycle. OXPHOS is regulated by cellular energy demand. Several factors tightly control the regulation of OXPHOS including the concentration of ATP and ADP, availability of oxygen, electron carriers (NADH and FADH2), and membrane potential. Tài liệu giúp bạn tham khảo, ôn tập và đạt kết quả cao. Mời bạn đón xem.
Môn: Writing BC2 1 tài liệu
Trường: Trường Đại học Quốc tế, Đại học Quốc gia Thành phố Hồ Chí Minh 696 tài liệu
Thông tin:
Tác giả:
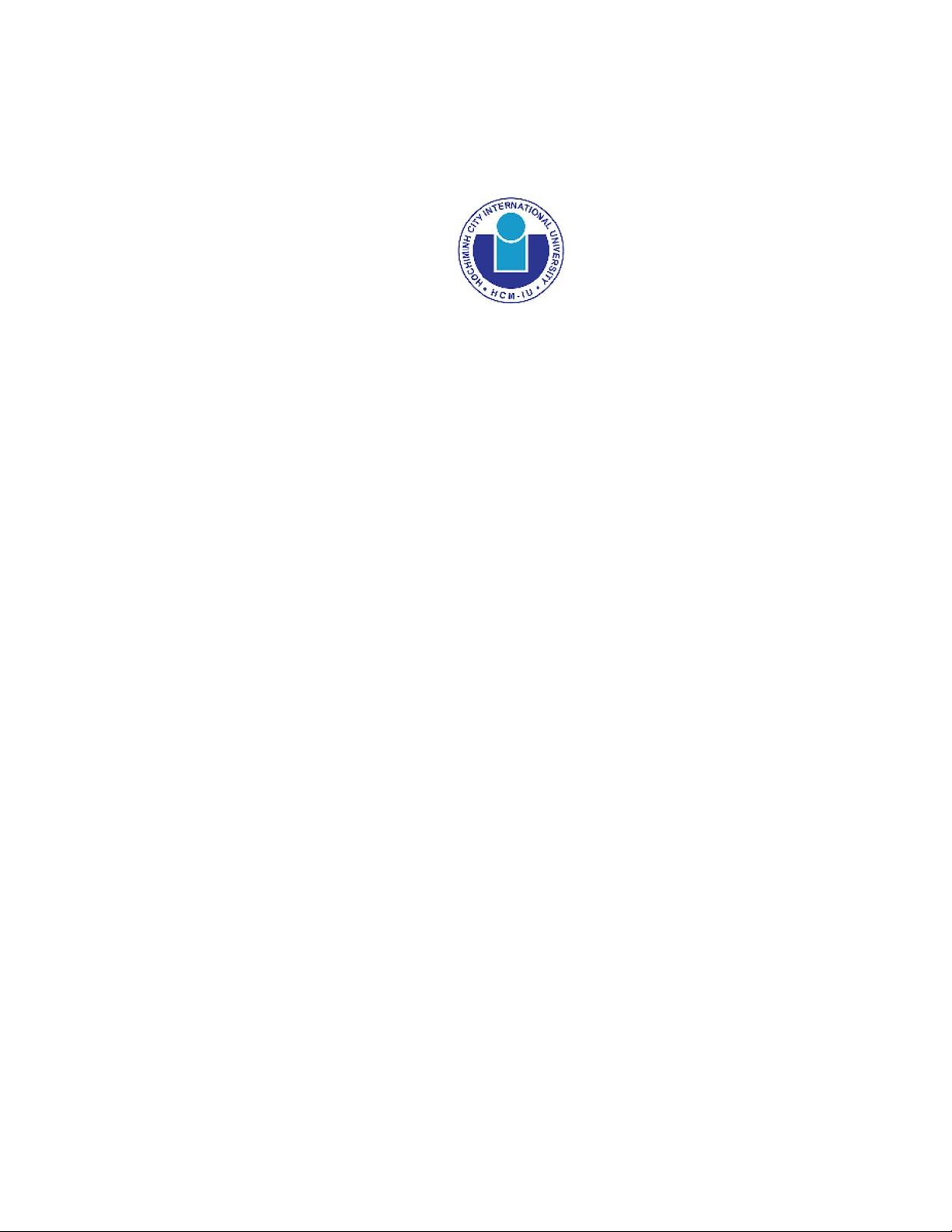
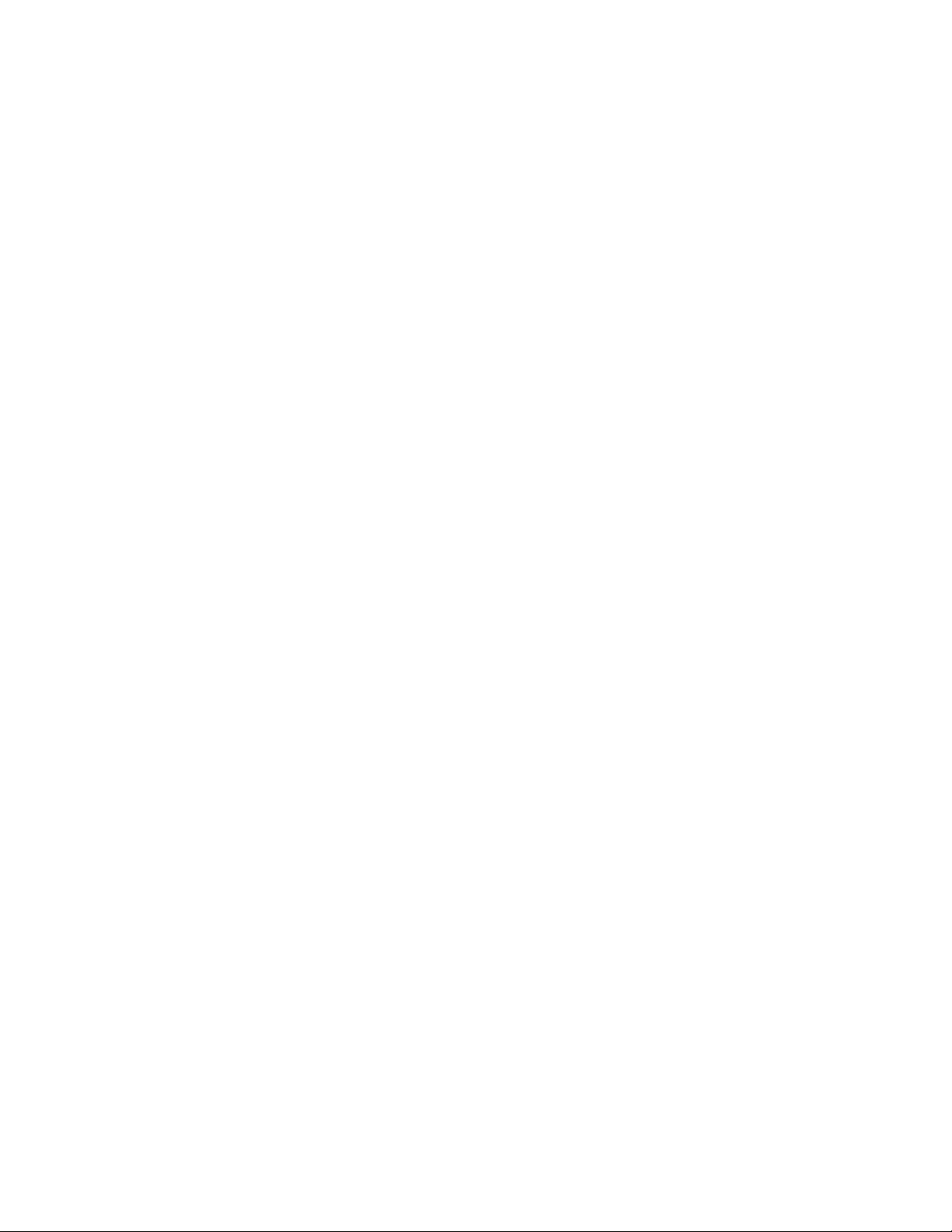
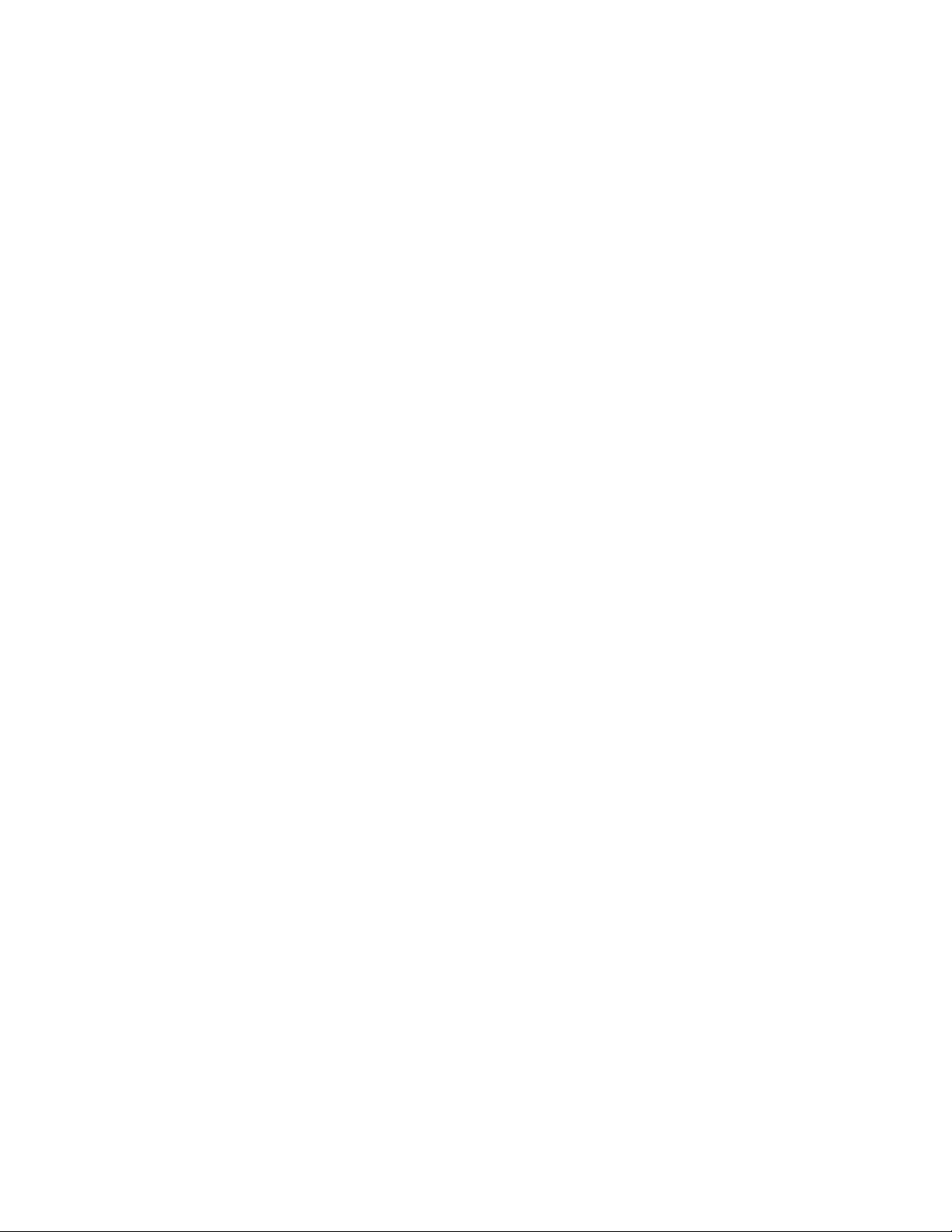
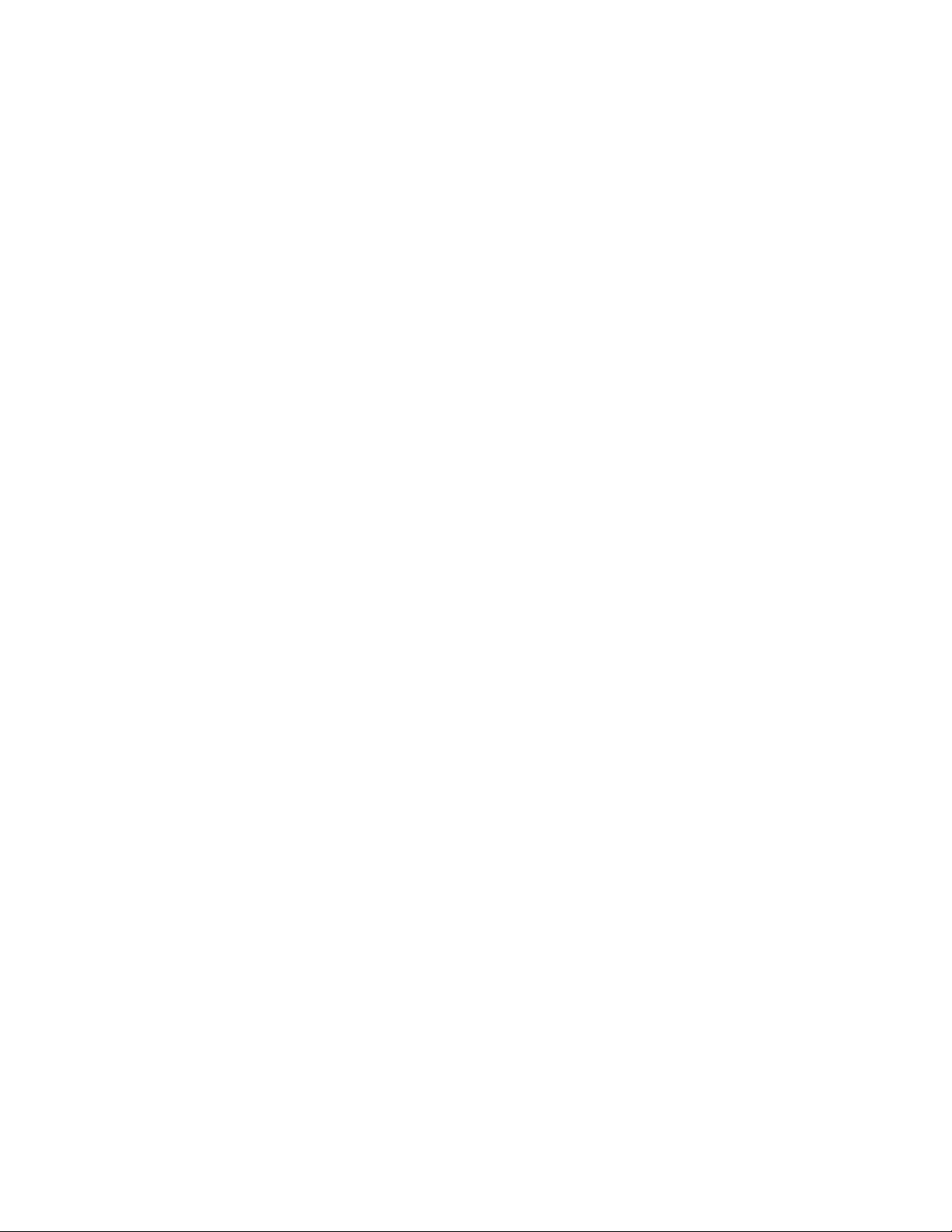
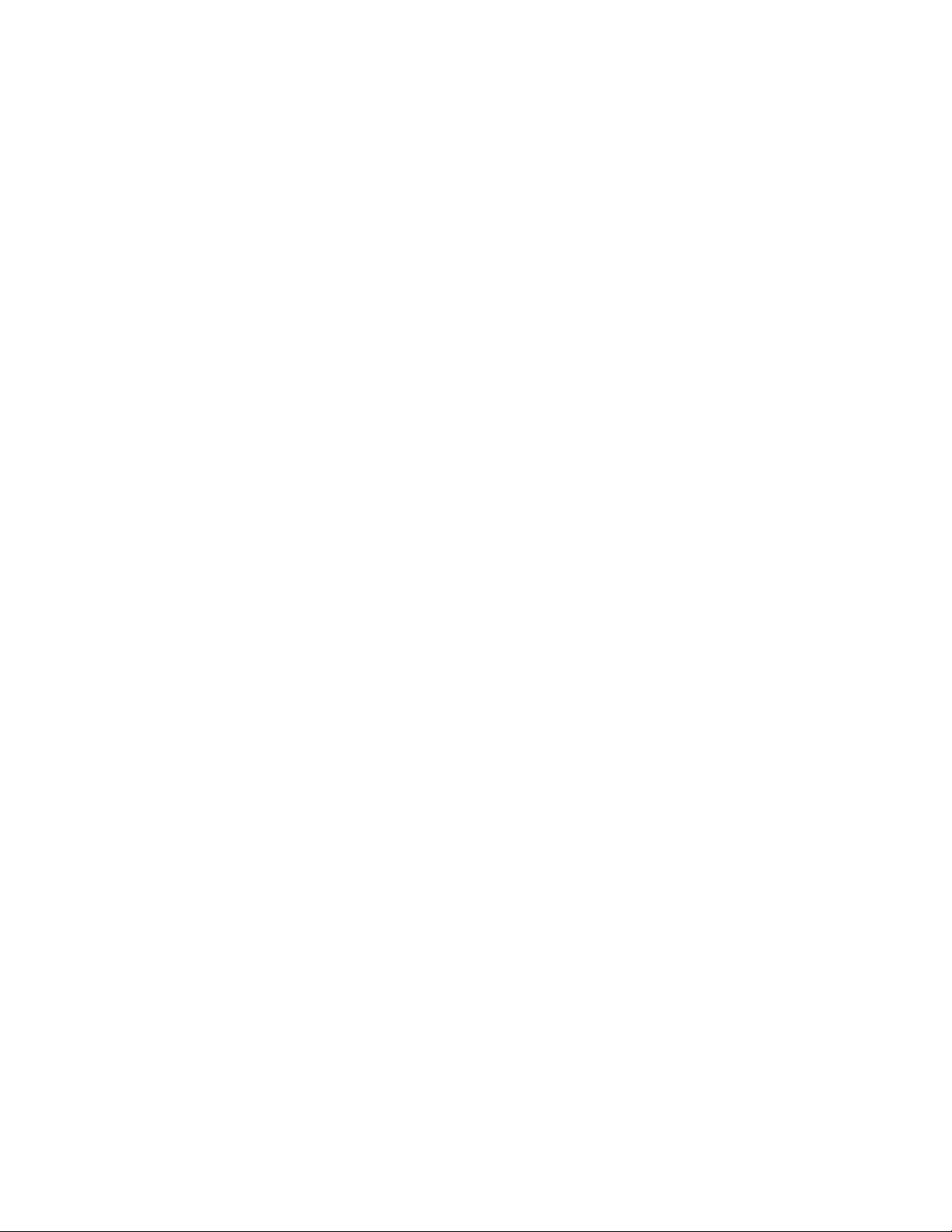
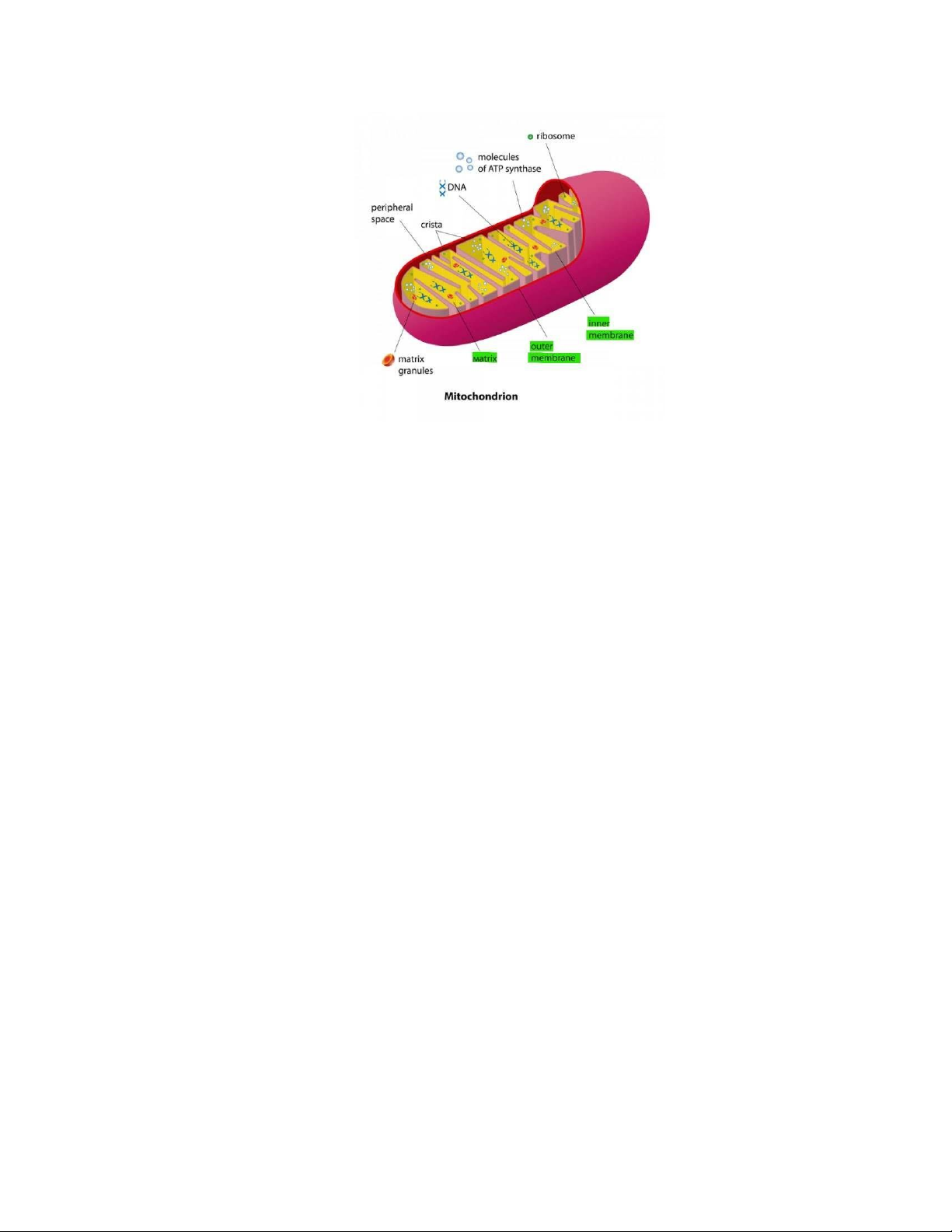
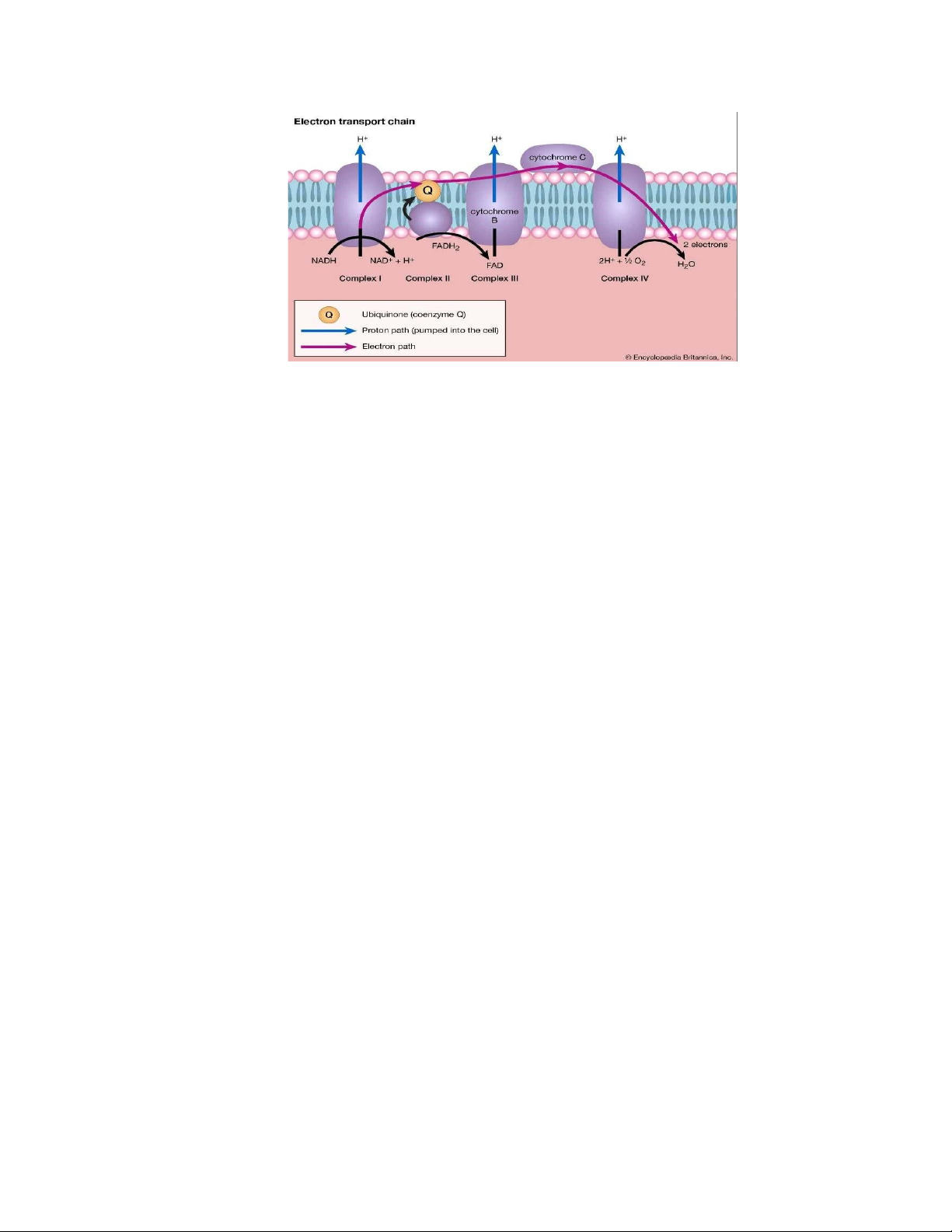
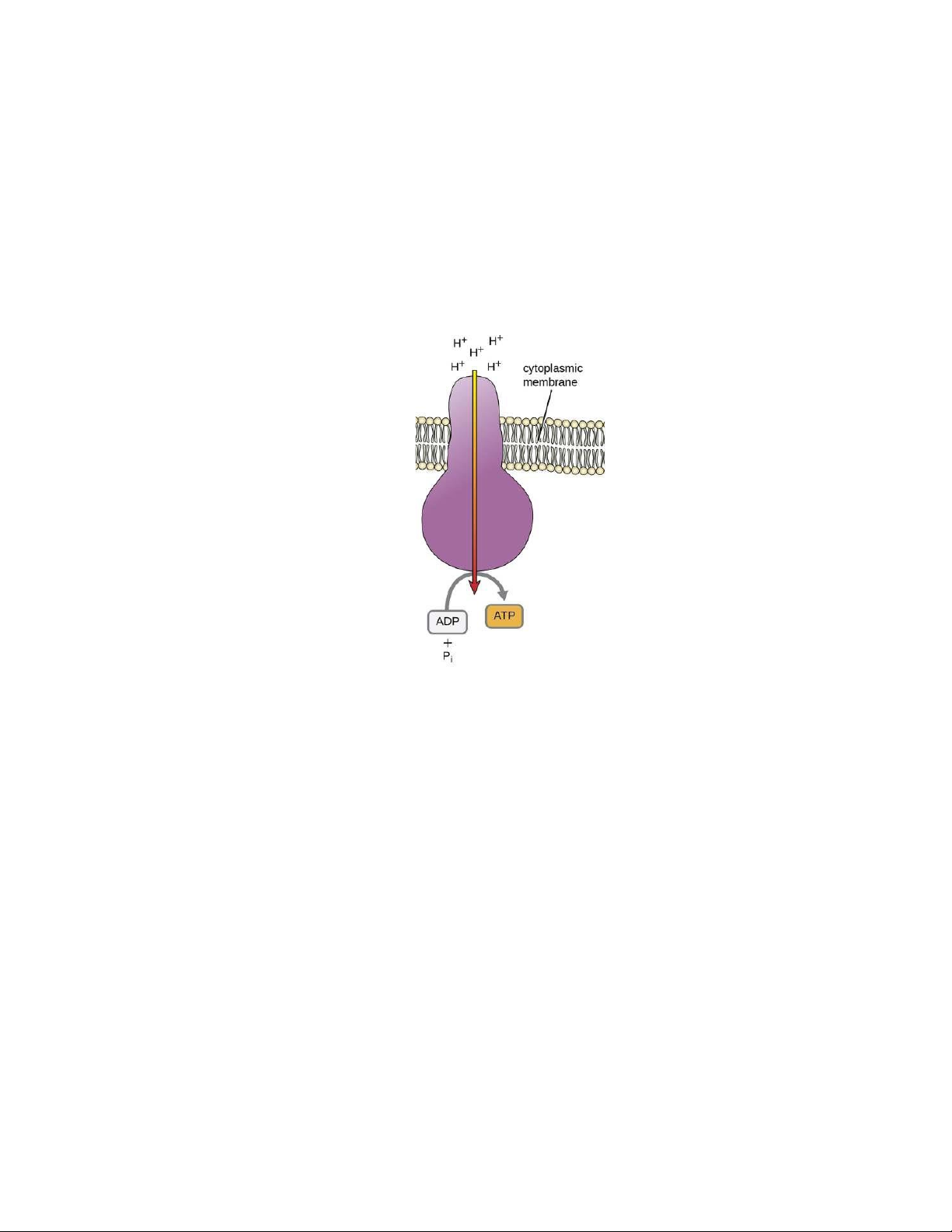
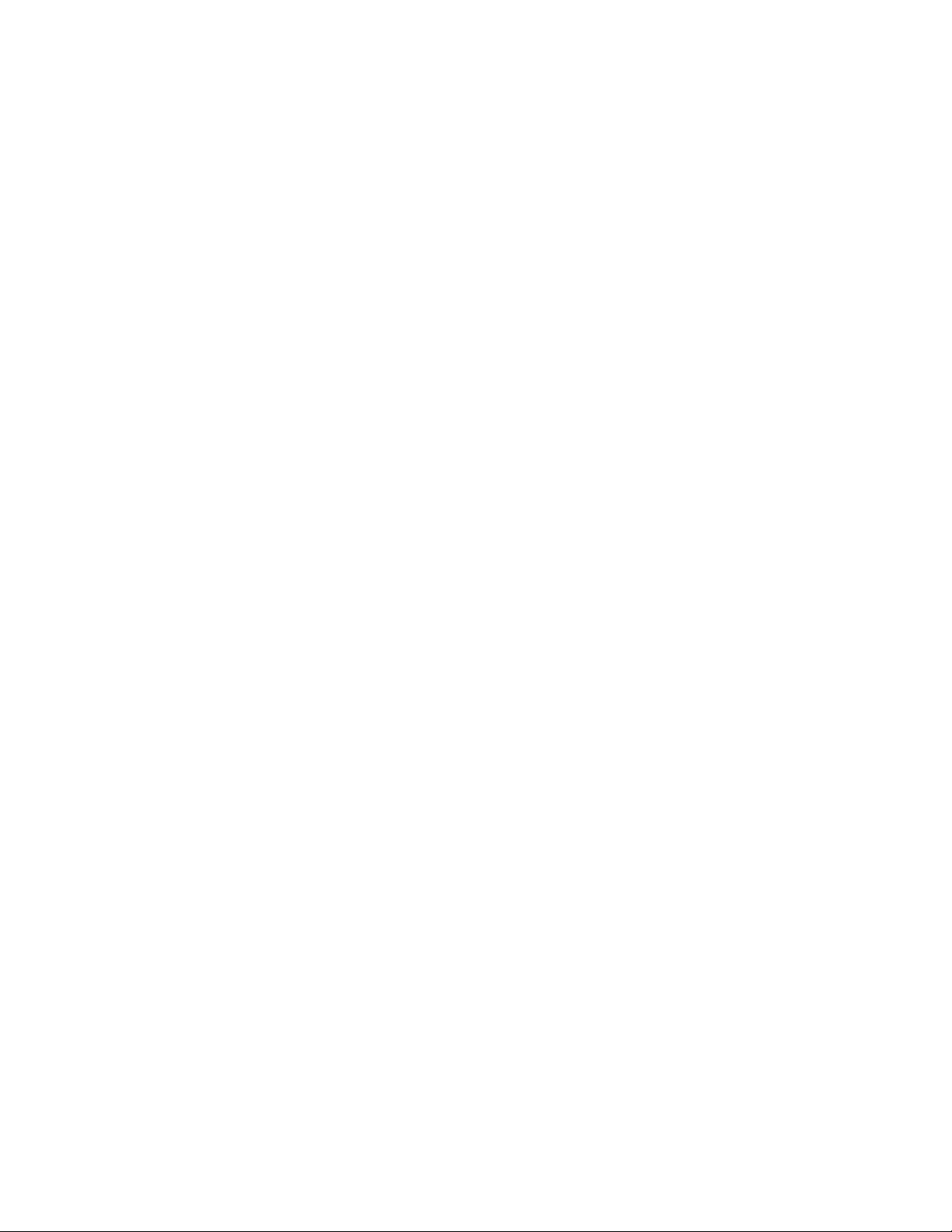
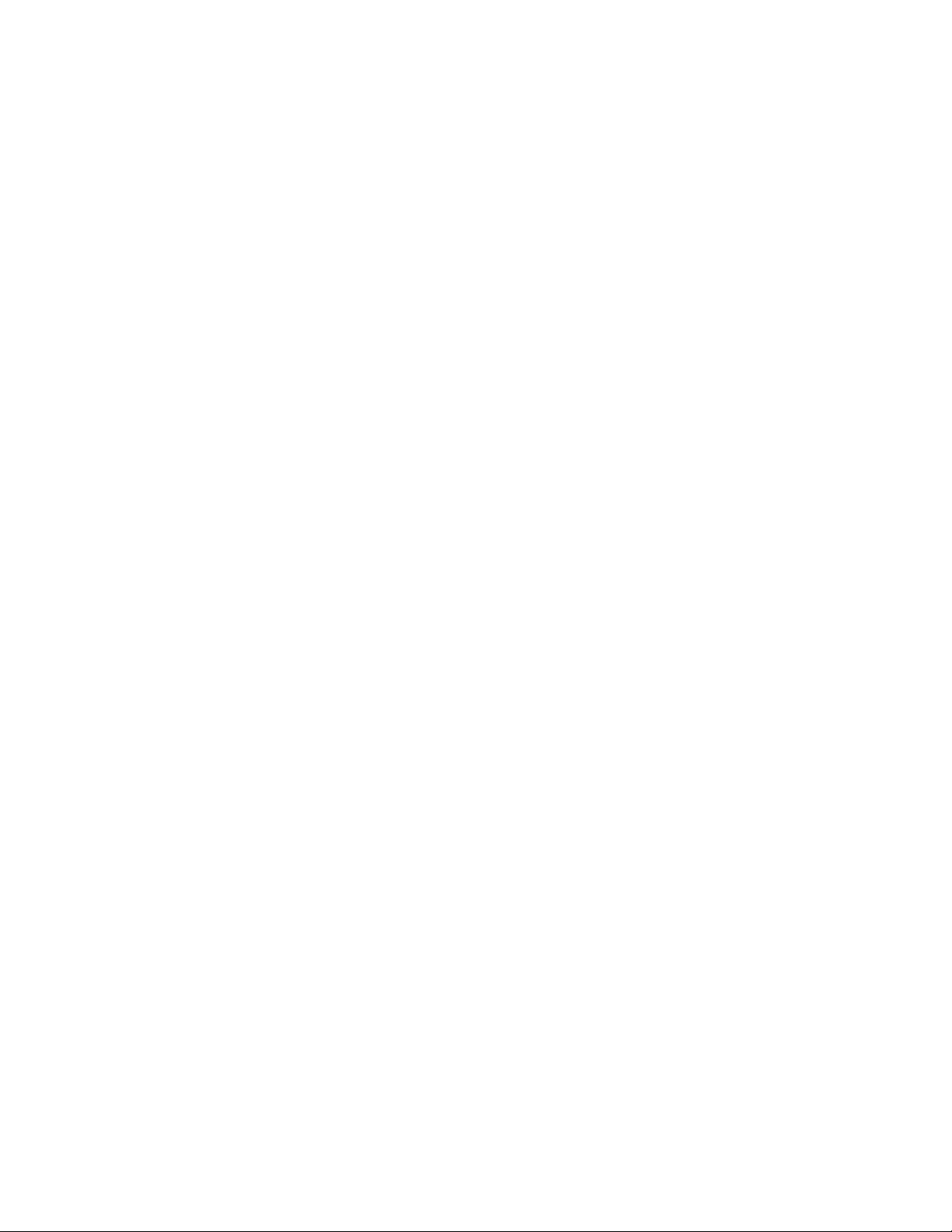
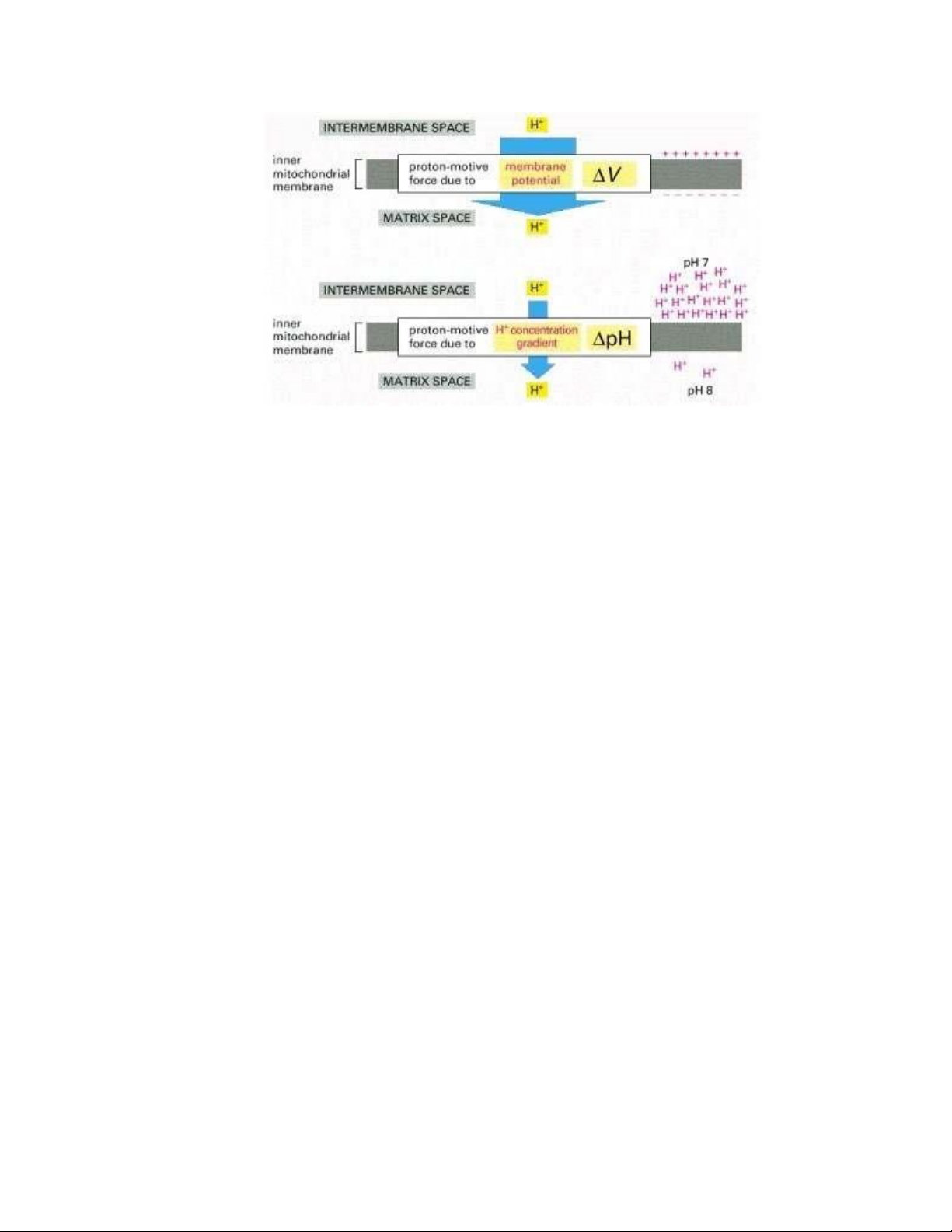
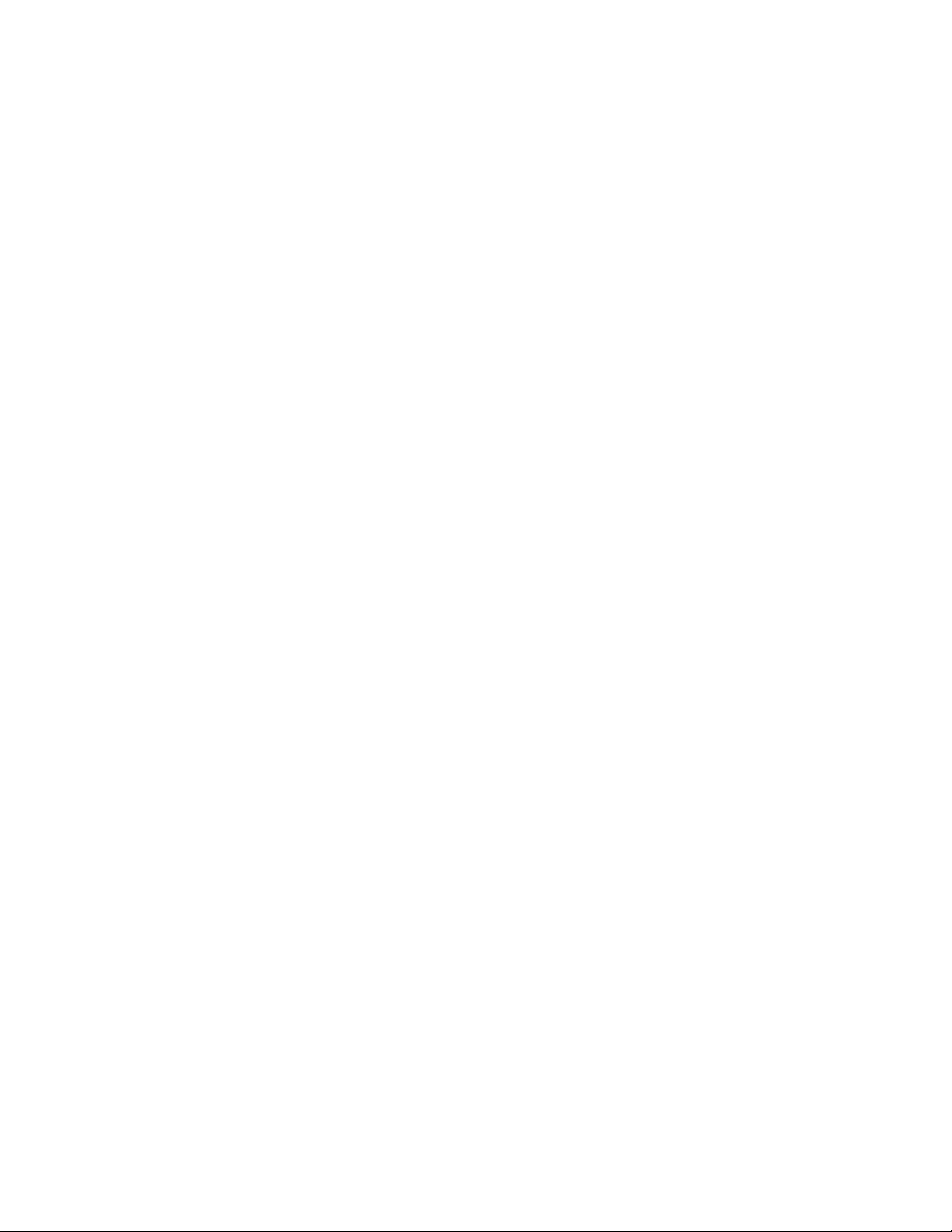
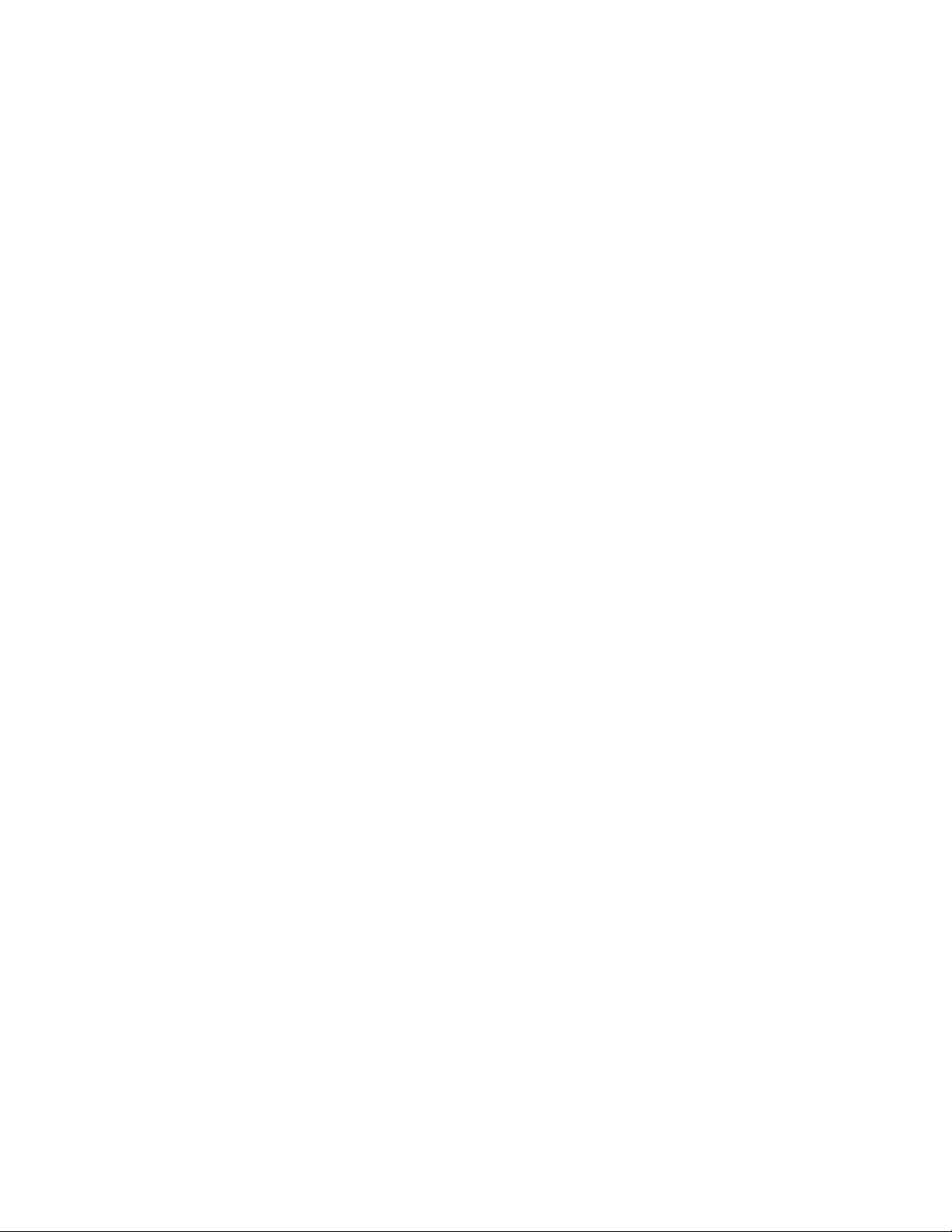
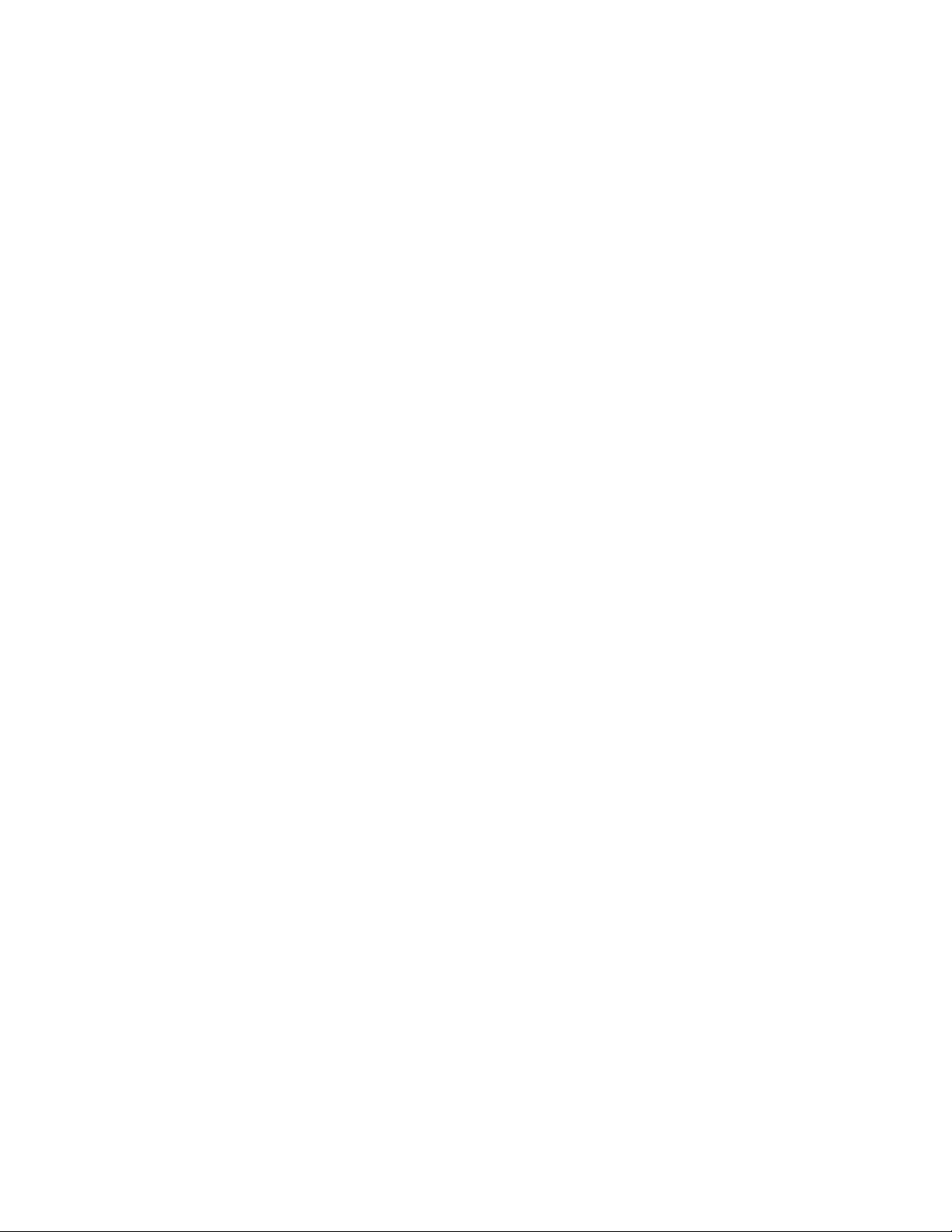
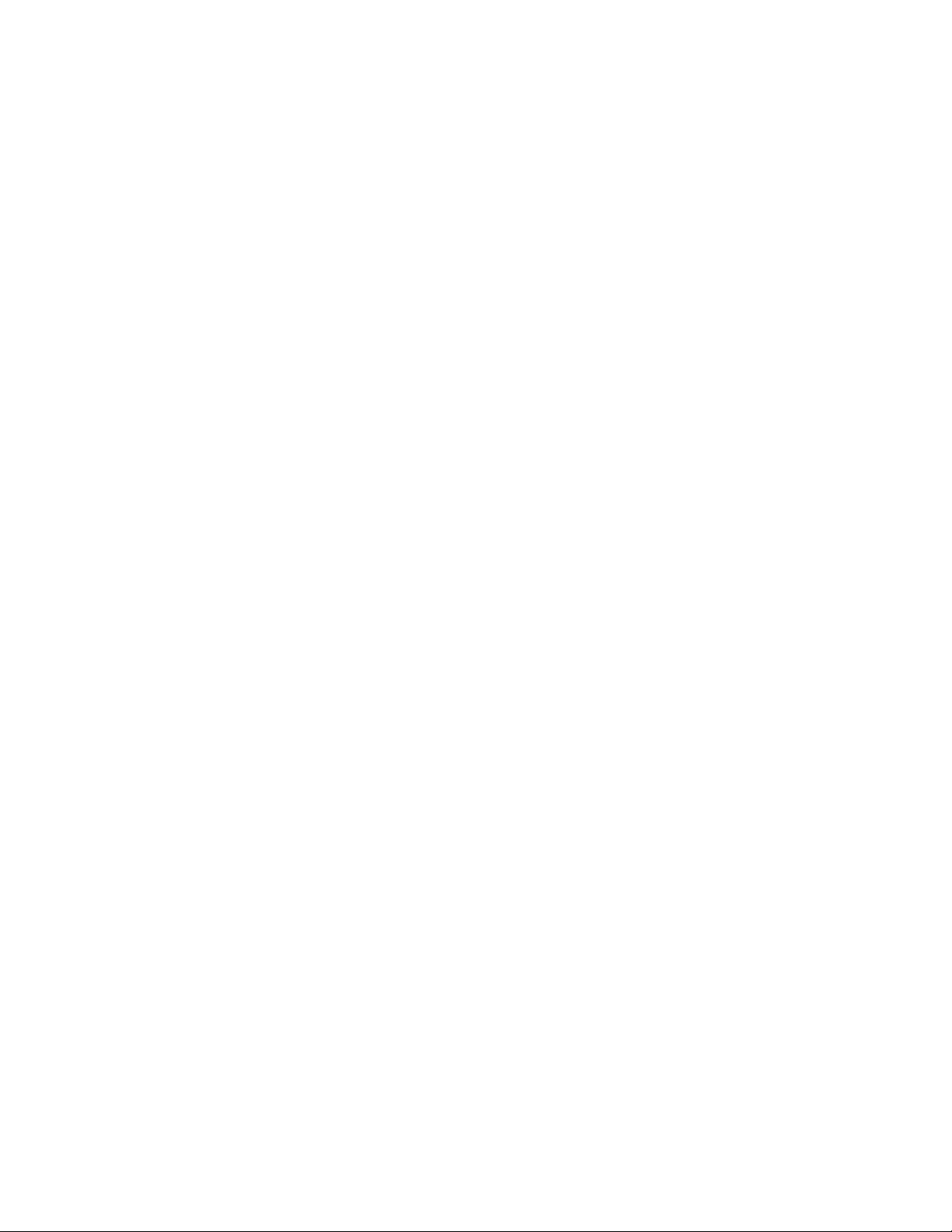
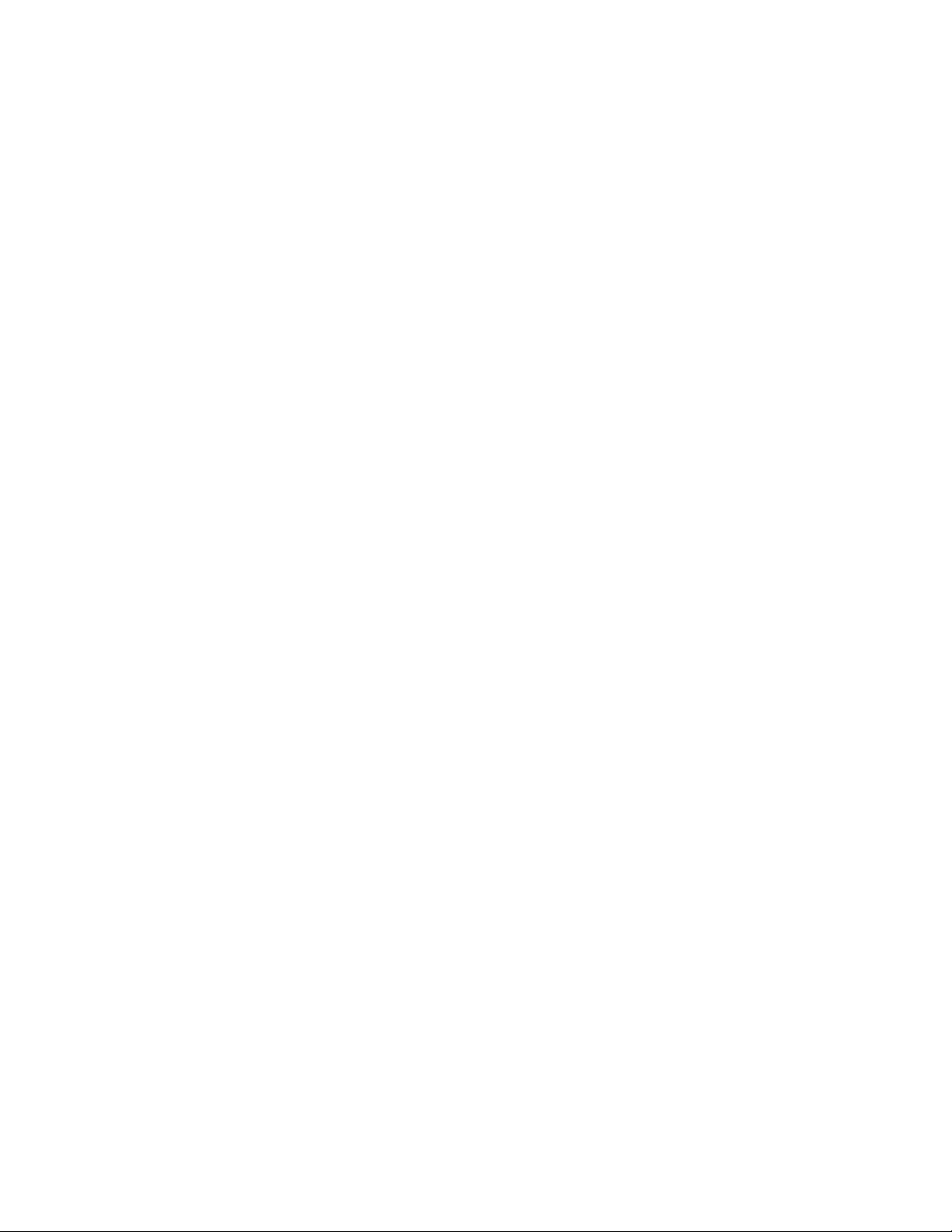
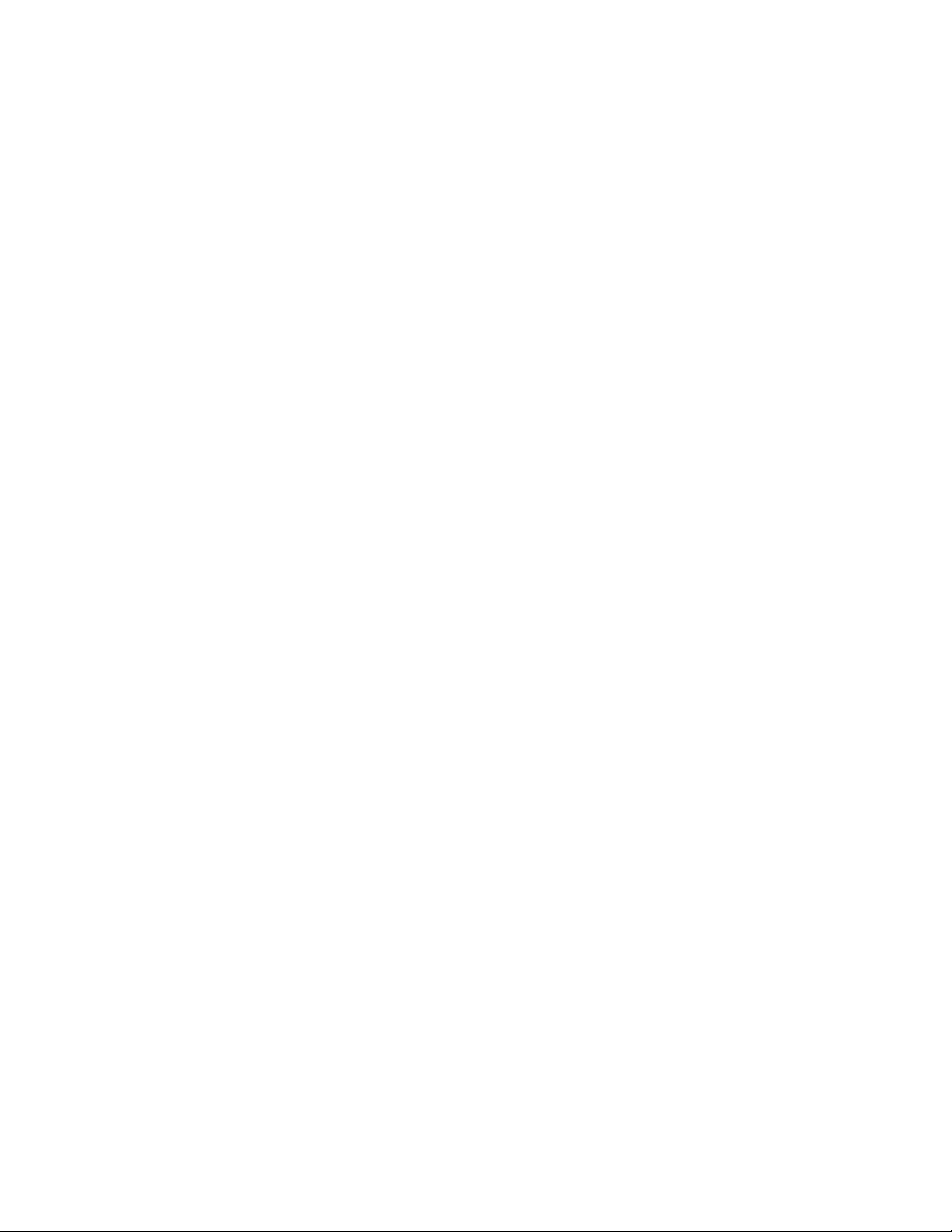
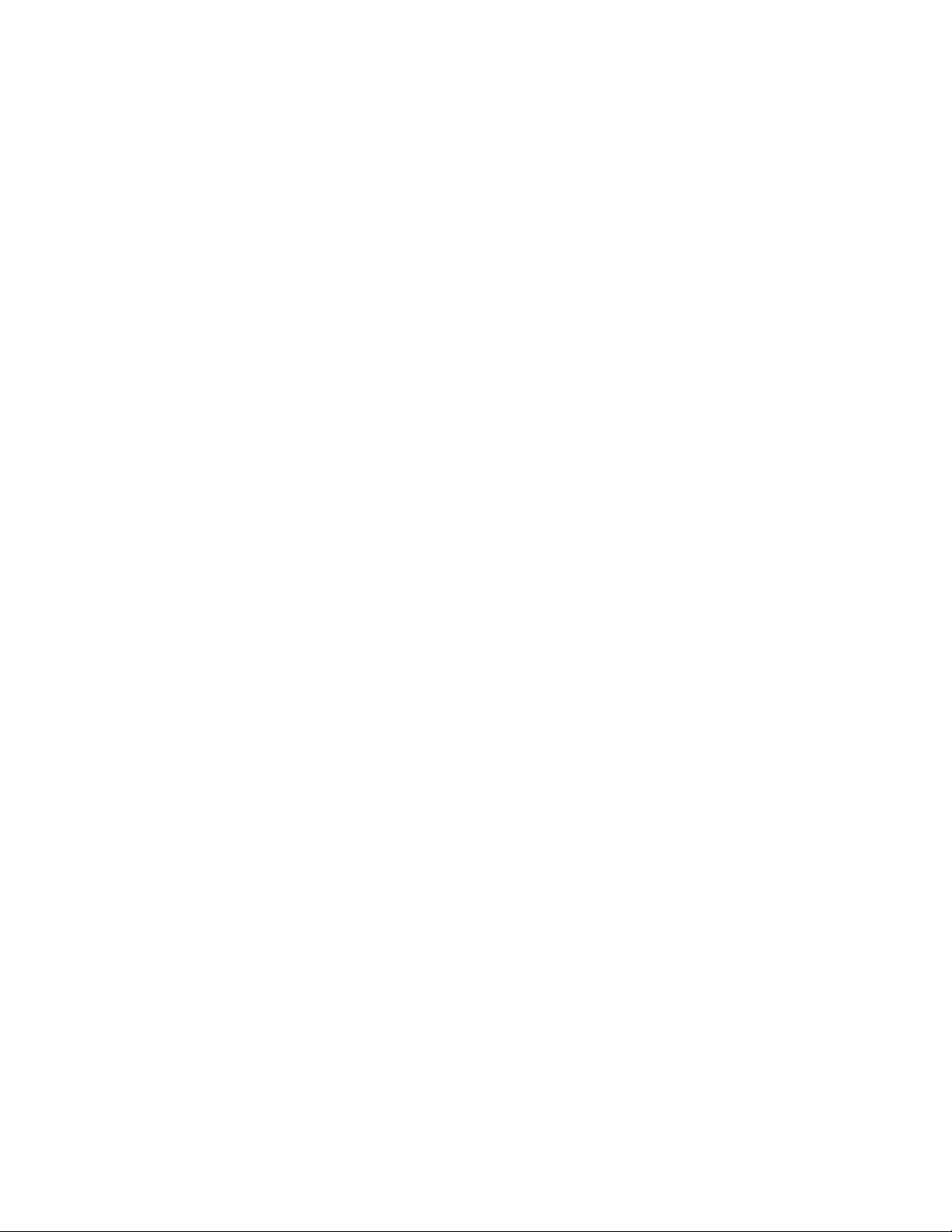
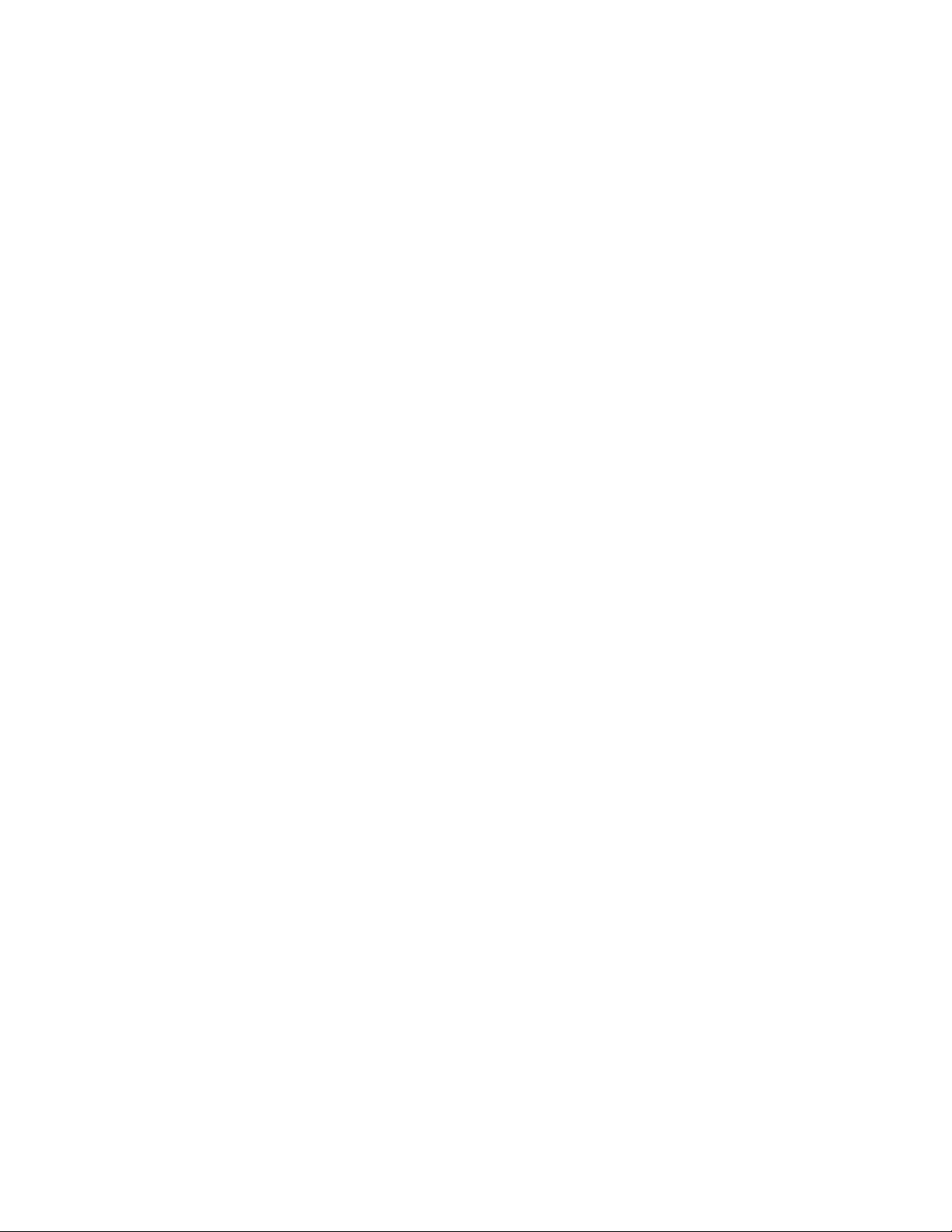
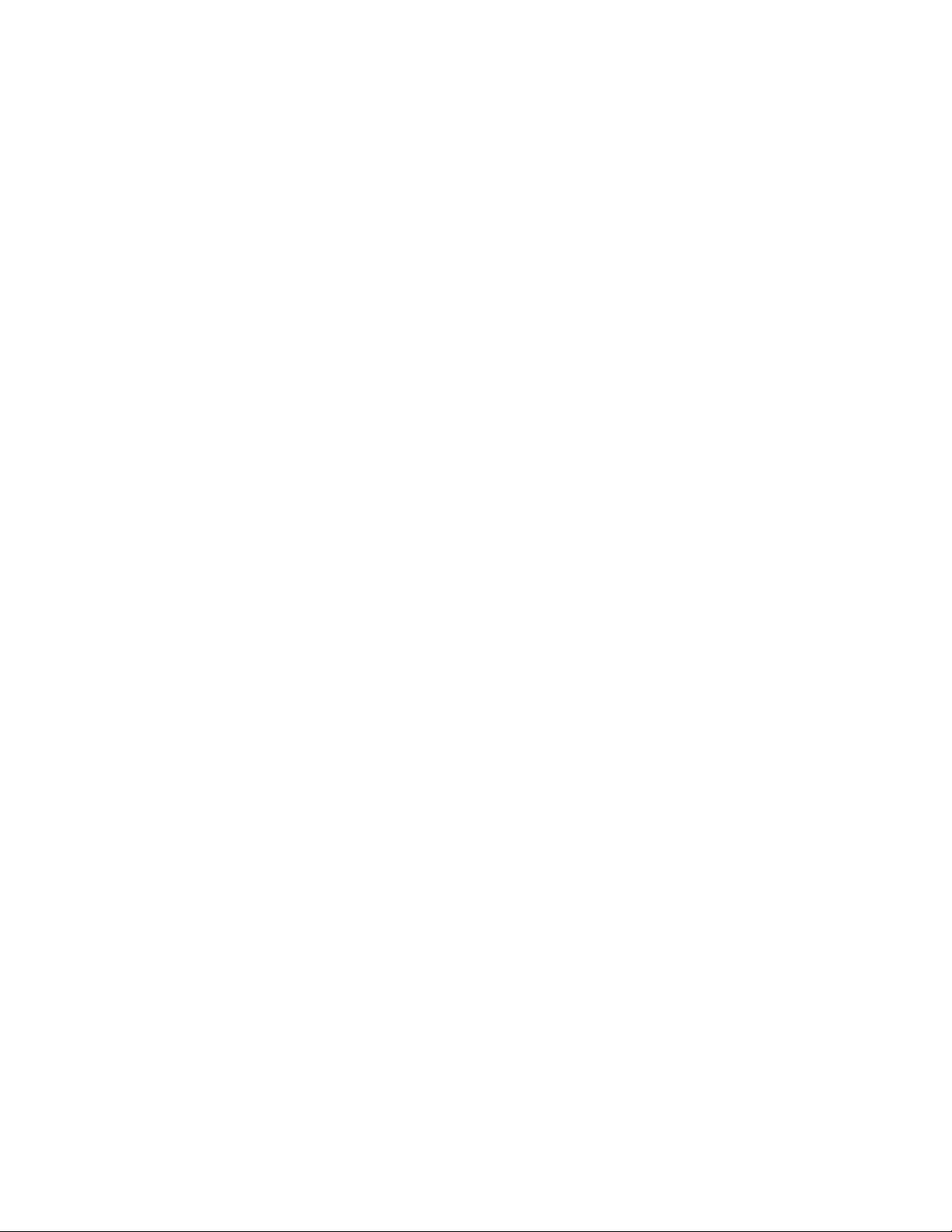
Tài liệu khác của Trường Đại học Quốc tế, Đại học Quốc gia Thành phố Hồ Chí Minh
Preview text:
VIETNAM NATIONAL UNIVERSITY - HO CHI MINH CITY
INTERNATIONAL UNIVERSITY
DYSREGULATION OF OXIDATIVE
PHOSPHORYLATION IN HUMAN DISEASES GROUP 8:
Lâm Gia Nghi BTBCIU18097 Phạm Mỹ Nhàn BTBCIU19054 Đào Thanh Trúc BTBCIU18096
INSTRUCTOR: Vũ Thanh Ngọc May 2023 ACKNOWLEDGEMENT
This literature review has been completed successfully with much support. We would like
to express our sincere appreciation to our instructor, Vũ Thanh Ngọc for assigning us the
topic “Dysregulation of Oxidative phosphorylation in human diseases, which help us
learn more new things and experience in research and academic writing. Her enthusiasm
and immense knowledge have inspired us to accumulate more knowledge and follow the
biochemistry field. Furthermore, we would like to make a special thanks to our family for
their unconditional support, nursing, and understanding. No written word can adequately
express our appreciation to all the people. We are delighted to get acquainted with you. TABLE OF CONTENTS
ABSTRACT ...................................................................................................................... 1
1. INTRODUCTION ....................................................................................................... 1
1.1. Overview of mitochondria ........................................................................... 1
1.2. Overview of oxidative phosphorylation ..................................................... 2
1.2.1. Definition of oxidative phosphorylation ..................................................... 2
1.2.2. Synthesis of ATP ......................................................................................... 4
2. REGULATION OF OXIDATIVE PHOSPHORYLATION .................................... 5
3. DYSREGULATION OF THE PATHWAY IN HUMAN DISEASES ..................... 8
3.1. Oxidative phosphorylation in the human body .......................................... 8
3.1.1. The oxidative phosphorylation (OXPHOS) pathway and its role in cells
and the human body ............................................................................................... 8
3.1.2. The interest of scientists in studying the abnormalities and dysregulation
of the OXPHOS pathway in diseases .................................................................... 8
3.2. Methods to detect abnormalities and dysregulation of the OXPHOS
pathway ................................................................................................................. 9
3.2.1. The method of analyzing the functional domain of the OXPHOS
pathway .................................................................................................................. 9
3.2.2. The method of analyzing genes ................................................................. 10
3.2.3. The method of analyzing proteins ............................................................. 11
3.2.4. The method of evaluating cellular functions and energy .......................... 12
4. CONCLUSION .......................................................................................................... 13
REFERENCES ............................................................................................................... 15 LIST OF FIGURES
Figure 1. Animation of mitochondria structure (Margarida Azevedo, 2015) .................. 2
Figure 2. The electron transport chain in the inner membrane of mitochondria (John P.
Rafferty, 2017) .................................................................................................................. 3
Figure 3. ATP synthase pumps protons back to generate ATP (Klaus Hoffmeier, 2020) 4
Figure 4. The two components of the electrochemical proton gradient (Alberts et al.,
2002). ................................................................................................................................ 7 LIST OF ABBREVIATIONS ADP Adenosine diphosphate ATP Adenosine Triphosphate ETC Electron transport chain FADH2 Flavin adenine dinucleotide FoF1-ATPase proton-pumping ATP synthase NADH
Nicotinamide adenine dinucleotide OXPHOS Oxidative phosphorylation PFK-1 phosphofructokinase-1 Pi Free phosphate SNPs Nucleotide polymorphisms TCA Tricarboxylic acid lOMoARcPSD|47231818
Dysregulation of Oxidative phosphorylation in human diseases
Lâm Gia Nghi a, Phạm Mỹ Nhàn a, Đào Thanh Trúc a a School of Biotechnology,
International University – Vietnam National University in HCMC ABSTRACT
Oxidative phosphorylation (OXPHOS) is the final stage of cellular respiration, which is
counted as the most abundant source of Adenosine Triphosphate (ATP) among the three
processes of cellular respiration. These three processes are Glycolysis, the Krebs cycle,
and Oxidative phosphorylation, respectively. The fundamental mechanism of oxidative
phosphorylation is to synthesize ATP based on accumulated proton via the electron
transport chain (ETC). Fumarate that is derived from this process can also enter the Krebs
cycle. OXPHOS is regulated by cellular energy demand. Several factors tightly control
the regulation of OXPHOS including the concentration of ATP and ADP, availability of
oxygen, electron carriers (NADH and FADH2), and membrane potential. The dysfunction
of oxidative phosphorylation is also mentioned. The method of analyzing the functional
domain of the OXPHOS pathway, genes, and proteins, and assessing cellular function and
energy that are utilized to detect abnormalities in the OXPHOS pathway are described in order. 1. INTRODUCTION
1.1. Overview of mitochondria
Mitochondria are the organelles inside animal and plant cells. It plays a significant role in
generating the energy known as ATP which provides cellular work. Two-over-three steps
of cellular respiration take place in the mitochondria: the Krebs cycle and oxidative phosphorylation (OXPHOS). 1
Figure 1. Animation of mitochondria structure (Margarida Azevedo, 2015)
The mitochondrion is one of three double-membrane organelles inside the cell. The inner
membrane has a phospholipid structure containing hydrophobic tails and hydrophilic
heads with many proteins embedded.
The OXPHOS discussed in this review mainly occurs in the inner membrane of
mitochondria. Besides, the intermembrane space is also involved as the container of protons.
1.2. Overview of oxidative phosphorylation
1.2.1. Definition of oxidative phosphorylation
OXPHOS is a cascade of redox reactions that occur in mitochondria. This is the final stage
of cellular respiration, which is counted for the most abundant ATP generated. It causes
the accumulation of protons in matrix space. Two components that build up a mechanism
are the electron transport chain and chemiosmosis. In the mechanism, the electron
transport chain is composed of four complexes marked as complex I-IV (proteins), and
organic molecules (Ubiquinone/Q/CoQ, and cytochrome c) are embedded in the inner
membrane of mitochondria. Chemiosmosis has the enzyme ATP synthase combined with
proton to produce ATP at the end. 2
Figure 2. The electron transport chain in the inner membrane of mitochondria (John P. Rafferty, 2017)
The first electron acceptor is Nicotinamide Adenine Dinucleotide (NADH) which is taken
from the other stage of cellular respiration. The second electron acceptor is Flavin Adenine
Dinucleotide (FADH2). In chemiosmosis, the final electron acceptor is oxygen which is
derived from inhalation. By that means, if the living organism does not intake oxygen
from the outside into the body, the electron transport chain will be stopped. It is considered
an aerobic process. OXPHOS involved three main steps including the delivery of electrons
by NADH and FADH2, the movement of electrons to complex III, and the reduction of oxygen into water (Figure 2).
NADH and FADH2 are oxidized at complex I and complex II, respectively, to give NAD+,
FAD+, and electrons. After that, the electrons will move to Ubiquinone Q which is a
mediate molecular in the system. A part of them acts as the energy for pumping proton
from the matrix to the intermembrane space against the gradient, called the proton-motive
force. In complex II, there is also the formation of fumarate derived from succinate that
can enter the Krebs cycle. Complex III is also involved in proton pumping, which forces
the proton H+ out of the matrix to intermembrane space. And the electrons keep moving
to the cytochrome c. After the electrons are passed through complex I/complex II to CoQ
to Complex III and to cytochrome C, it arrives complex IV at which steps the oxygen will
receive the electrons to reduce into oxygen ion. The oxygen ion then combines with the proton to form water. 3 1.2.2. Synthesis of ATP
Proton moves back from intermembrane space into the matrix to combine with ATP
synthase and make ATP. However, protons cannot pass through the hydrophobic tail of the
membrane. The ATP synthase provides a canal for the proton to move in the matrix. The
proton is a catalyst to add the phosphate (Pi) to Adenosine Diphosphate (ADP) to become
ATP in a phosphorylation reaction.
Figure 3. ATP synthase pumps protons back to generate ATP (Klaus Hoffmeier, 2020)
Because the OXPHOS pathway is a crucial process for energy production, abnormal
OXPHOS can lead to health problems and energy-related diseases. In this review, the
regulation of the OXPHOS pathway is discussed initially. Several factors tightly control
the regulation of OXPHOS that are mentioned including the concentration of ATP and
ADP, availability of oxygen, electron carriers (NADH and FADH2), and membrane
potential. To detect the abnormalities and dysregulation of the OXPHOS pathway, the
method of analyzing the functional domain of the OXPHOS pathway, genes, and proteins,
and assessing cellular function and energy are described in order. 4
2. REGULATION OF OXIDATIVE PHOSPHORYLATION
Aerobic oxidative pathways that lead to electron transfer to oxygen are accompanied by
OXPHOS. OXPHOS provides tremendous energy efficiency of catabolism since it
produces more than 15 times the ATP generated by anaerobic glycolysis (Alberts et al.,
2002). Therefore, it is crucial to regulate ATP production by OXPHOS to match the cell’s
fluctuating demand for ATP. The OXPHOS is regulated by several factors including
concentration of ADP and ATP, availability of oxygen, electron carriers (NADH and
FADH2), and membrane potential.
In tight regulation, the rate of respiration in mitochondria is generally limited by the
availability of ADP as a phosphorylation substrate. A measure of the energy state of cells
is the intracellular concentration of ADP. The mass-action ratio of the ATP-ADP system,
[ATP]/([ADP][Pi]), is another related measure. Normally, this ratio is quite high for the
almost full phosphorylation of the ATP-ADP system. The rate of ATP breakdown into
ADP and Pi increases as the rate of any energy-intensive process, such as protein synthesis,
results in a lowering in the mass-action ratio. The rate of respiration increases as there is
more ADP available for OXPHOS, which results in ATP regeneration. This continues until
the mass-action ratio returns to its normal high level, at which point respiration slows
again. Even with significant fluctuations in energy demand, the [ATP]/([ADP][Pi]) ratio
in most tissues fluctuates slightly because the rate of oxidation of cellular fuels is regulated
with such sensitivity and precision. In other words, ATP is only produced fast as it is used
in energy-requiring cellular activities.
ATP synthase catalyzes the reverse of ATP synthesis as an ATP-driven proton pump. When
a cell is deprived of oxygen, electron transfers to oxygen and the pumping of protons
ceases leading to the proton-motive force collapsing. Additionally, the main source of ATP
becomes glycolysis, and the pyruvic or lactic acid thus formed lowers the pH in the cytosol
and the mitochondrial matrix. Under these conditions, the ATP synthase could operate in
reverse, hydrolyzing ATP to pump protons outward and causing a disastrous drop in ATP
levels. This is prevented by a small (84 amino acids) protein inhibitor, IF1. At a pH lower
than 6.5, IF1 inhibits the ATPase activity in its dimeric form. It binds to the ab interface
of the subunits in the diphosphate (ADP) conformation (αADP and βADP), freezing the 5
two F1 complexes and thereby blocking ATP hydrolysis. When aerobic metabolism
resumes, production of pyruvic acid slows, the pH of the cytosol rises, the IF1 dimer is
destabilized, and the inhibition of ATP synthase is lifted.
ETC is in the inner mitochondrial membrane that is responsible for generating a proton
gradient across the membrane. This gradient is then used to drive the synthesis of ATP
through OXPHOS. During OXPHOS, NADH and FADH2 transfer electrons to the ETC,
which then uses the energy from these electrons to pump protons across the inner
mitochondrial membrane, creating an electrochemical gradient that drives ATP synthesis
through chemiosmosis. NADH and FADH2 have different effects on OXPHOS. NADH
delivers electrons to complex I of the ETC, which pumps more protons than complex II
and therefore contributes more to the proton gradient and ATP synthesis. FADH2 delivers
electrons to complex II, which does not pump as many protons as complex I, resulting in less ATP production.
As mentioned above, protons are pumped across the inner mitochondrial membrane as
electrons pass through the electron transfer chain creating a proton gradient. This
electrochemical gradient includes contributions from both a membrane potential and a pH
gradient. The pH gradient drives H+ return to the matrix and OH- out of the matrix,
thereby reinforcing the effect of the membrane potential, which acts to attract any positive
ion into the matrix and push any negative ion out (Figure 4). A large amount of free energy
is released that provides the basis for ATP production in the matrix. The effective ATPADP
exchange between the mitochondrion and the cytosol, which maintains the cell's ATP pool
in a highly charged state, is one of the active transport processes that are driven by the
transmembrane electrochemical gradient. The high ratio of ATP to its hydrolysis products
that results makes the free-energy change for ATP hydrolysis exceedingly favorable,
allowing this hydrolysis reaction to drive a significant portion of the cell's energy- consuming functions. 6
Figure 4. The two components of the electrochemical proton gradient (Alberts et al., 2002).
The primary sources of the electrons that enter the mitochondrial electron-transport chain
are glycolysis, fatty acid degradation, and the citric acid cycle. These pathways have
interlocking and concerted regulatory mechanisms that allow them to function together in
an economical and self-regulating manner to produce ATP and biosynthetic precursors. It
can be seen that the control of glycolysis and the citric acid cycle is coordinated with the
demand for OXPHOS. The rate of electron transfer and OXPHOS increases whenever
ATP consumption increases. The influx of electrons into the respiratory chain also rises
simultaneously with an increase in the rate of pyruvate oxidation via the citric acid cycle.
These events can in turn evoke a rise in glycolysis, which would increase the rate of
pyruvate synthesis. Acceptor control inhibits electron transport when the conversion of
ADP to ATP reduces the ADP concentration, and thus OXPHOS. Because ATP is an
allosteric inhibitor of the glycolytic enzyme phosphofructokinase-1 (PFK-1), glycolysis
and the citric acid cycle are also slowed. The first intermediate of the citric acid cycle,
citrate, also inhibits PFK-1. Citrate builds up inside mitochondria and overflows into the
cytosol when the cycle is "idling," (Lehninger et al., 2008). A concerted allosteric
inhibition of PFK-1 is produced when the levels of ATP and citrate both increases. This
inhibition is higher than the sum of the separate effects and slows glycolysis. 7
3. DYSREGULATION OF THE PATHWAY IN HUMAN DISEASES
3.1. Oxidative phosphorylation in the human body
3.1.1. The oxidative phosphorylation (OXPHOS) pathway and its role in cells and the human body
The OXPHOS pathway is the process of producing ATP by using the energy released from
the oxidation of organic compounds in the cells of the body. This process occurs in the
inner membrane of mitochondria within the cell. In the OXPHOS pathway, NADH and
FADH2 molecules produced in the TCA cycle are transferred to the mitochondrial ETC
and release electrons. These electrons move through a series of complexes (I, II, III, and
IV) in the ETC, creating potential electron energy. This electron energy pumps protons
(H+) from the mitochondrial matrix to the inner membrane, creating a higher proton
concentration outside the inner membrane. The concentration of protons creates an
electrochemical gradient, and the ATP synthase enzyme uses this energy to produce ATP from ADP and Pi.
The OXPHOS pathway provides energy for basic cellular processes, including the
conversion and synthesis of organic compounds, ion transport, and driving chemical
reactions. The OXPHOS process is also related to energy conversion in the body,
especially in maintaining body temperature and organ function. In addition, pathologies
related to the OXPHOS pathway can cause health problems, including genetic and cardiovascular diseases.
3.1.2. The interest of scientists in studying the abnormalities and dysregulation
of the OXPHOS pathway in diseases
The OXPHOS pathway is an important cellular process for energy production. However,
when the OXPHOS pathway is abnormal or dysregulated, it can lead to energy-related
diseases such as diabetes, cardiovascular disease, Parkinson's disease, and depression.
Therefore, researching abnormalities and dysregulation of the OXPHOS pathway is very
important and has attracted the attention of many scientists. 8
Scientists are studying factors that affect the OXPHOS pathway, such as genes, proteins,
bacteria, and cells. They are also looking for treatment methods for diseases related to
abnormalities and dysregulation of the OXPHOS pathway.
Recent research has identified factors that can modulate the OXPHOS pathway and
improve energy-related diseases. For example, certain foods and nutrients have been
shown to affect the OXPHOS pathway. In addition, treatment methods for diabetes,
cardiovascular disease, and depression are also being developed based on new knowledge
about the OXPHOS pathway. In summary, abnormalities, and dysregulation of the
OXPHOS pathway are important and promising research topics for scientists. New
findings in this field may provide new methods to treat energy-related diseases and
improve the quality of life for humans.
3.2. Methods to detect abnormalities and dysregulation of the OXPHOS pathway
3.2.1. The method of analyzing the functional domain of the OXPHOS pathway
The method of analyzing the functional domain of the OXPHOS pathway is a technique
that analyzes the activities and functions of molecules and structures related to the
OXPHOS system in cells. This method uses modern tools and techniques such as
proteomics, transcriptomics, metabolomics, and cell electrochemistry to measure changes
in the functional domain of the OXPHOS system. The process of analyzing the functional
domain of the OXPHOS pathway includes five main steps, which are (1) measuring the
activity of electron complexes that analyzing the activity of electron complexes using
spectroscopic or cell electrochemistry methods to determine changes in their activity; (2)
analyzing protein and gene concentrations for measuring protein and gene concentrations
to detect changes in the functional domain of the OXPHOS system; (3) evaluating ATP
productivity and oxygen consumption which can measure ATP productivity and oxygen
consumption to determine the efficiency of the OXPHOS system; (4) analyzing the ability
to metabolize by using metabolomics to measure changes in intermediate metabolites in
the OXPHOS process; and (5) evaluating interactions between molecules and structures
through using proteomics methods to determine interactions between molecules and
structures in the OXPHOS system. Furthermore, the method of analyzing the functional 9
domain of the OXPHOS pathway is an important tool for understanding the activity of the
OXPHOS system and how it relates to health issues.
However, the functional domain analysis method for detecting abnormalities and
dysregulation of the OXPHOS pathway still has both advantages and limitations. For its
advantages, it can provide information about the activity of components of the OXPHOS
pathway. Specifically, functional domain analysis allows the determination of the function
of components of the OXPHOS pathway, such as ATP production systems and oxidative
and reductive processes involved in energy generation. Moreover, it also can enable the
detection of gene and protein mutations which can detect gene and protein mutations
related to OXPHOS by measuring their activity in the pathway. Lastly, functional domain
analysis allows the assessment of the effects of environmental factors and drugs on the
activity of the OXPHOS pathway. On the other hand, the functional domain analysis
method still gains drawbacks, which requires the use of complex techniques such as
measuring intermediate compound concentrations to determine the activity of components
in the OXPHOS pathway. In addition, to obtain accurate results, functional domain
analysis must be performed by experts with experience in this field, and the cost of this
method is relatively high, so it is not always widely used in practice.
3.2.2. The method of analyzing genes
The method of gene analysis is a technique for analyzing changes in DNA related to the
OXPHOS pathway. It allows for the detection of abnormalities and regulatory imbalances
in the expression of genes encoding proteins involved in OXPHOS. This method can be
performed in several ways, including (1) Whole genome sequencing will analyse the
entire genome sequence to identify variations in genes related to the OXPHOS system.
This is an expensive and time-consuming method, but it can detect rare or unclear
variations; (2) Gene fragment analysis which evaluates gene fragments related to the
OXPHOS system to identify variations such as single nucleotide polymorphisms (SNPs)
or larger variations such as insertions or deletions. This method is faster and cheaper than
whole genome sequencing, or (3) Gene expression analysis determines regulatory
imbalances in the production of proteins related to the OXPHOS system. This method can 10
be performed using tools such as microarrays or RNA sequencing to measure the expression levels of genes.
The results of gene analysis can help identify variations in genes related to OXPHOS,
thereby detecting abnormalities and regulatory imbalances in the OXPHOS system. Gene
analysis also allows researchers to assess the impact of these variations on the activity of
the OXPHOS system and provide solutions to improve the health status of cells and the body.
This method provides a comprehensive understanding of the genetic factors involved in
the OXPHOS pathway, which is essential for developing effective treatments and
therapies for related diseases. Moreover, it allows for the identification of specific genetic
mutations that may cause abnormalities in the OXPHOS pathway, thereby enabling early
detection and intervention before the onset of severe symptoms. The method is also highly
sensitive and can detect even minor changes in gene expression or activity, providing
valuable insights into the underlying mechanisms of the pathway. Besides these benefits,
the method may be limited by its inability to account for non-genetic factors that may
influence the OXPHOS pathway, such as environmental factors or epigenetic
modifications. In addition, the interpretation of results may be complicated by the complex
interactions between multiple genes and pathways involved in the OXPHOS system.
Overall, while the method of analyzing gene function has its advantages and limitations,
it remains an essential tool for investigating the underlying genetic factors contributing to
abnormalities and imbalances in the OXPHOS pathway.
3.2.3. The method of analyzing proteins
The protein analysis method is a technique that allows for the analysis of changes in
proteins related to the OXPHOS pathway. It enables the detection of abnormalities and
regulatory imbalances in the production of proteins related to OXPHOS. There are three
major ways to perform this technique, which are (1) Western blotting; (2)
Immunoprecipitation; and (3) Mass spectrometry. In Western blotting, this method allows
for the analysis of the level of a specific protein by using a specific antibody for that
protein. These techniques provide information on the level and size of the protein, while 11
immunoprecipitation allows for the separation of a specific protein from a protein mixture
using a specific antibody. Once the protein has been separated, it can be used to analyze
the different parameters of that protein in detail. Through mass spectrometry, it requires
the analysis of the protein composition of a sample by measuring the mass of each
individual protein. This is a powerful method and is widely used in protein and energy
metabolism research. In conclusion, the results of protein analysis can help identify
abnormalities and regulatory imbalances in the production of proteins related to the
OXPHOS pathway. This method also allows researchers to assess the impact of these
changes on the activity of the OXPHOS system and provide solutions to improve the
health status of cells and the body.
The method provides direct information on the expression and activity of proteins
involved in the OXPHOS pathway, which can help identify potential targets for
therapeutic interventions. Furthermore, it can be used to identify post-translational
modifications of proteins, such as phosphorylation or acetylation, which can provide
insights into the regulation of the pathway. This method is highly specific, which allows
for the identification of individual protein isoforms and their expression levels. In contrast,
this technique still has some disadvantages. First, it may be limited by the availability and
quality of samples, which can be affected by factors such as sample collection, storage,
and processing. Secondly, it may be challenging to isolate and quantify specific proteins
of interest from complex biological samples, such as tissue or blood. Next, the
interpretation of results can be challenging due to the complexity of the OXPHOS
pathway and the interactions between different proteins and pathways involved.
Overall, while the method of protein analysis has its advantages and limitations, it remains
a valuable tool for investigating the expression and activity of proteins involved in the
OXPHOS pathway and identifying potential targets for therapeutic interventions.
3.2.4. The method of evaluating cellular functions and energy
Assessing cellular function and energy is a common method to detect abnormalities and
dysregulation in the OXPHOS pathway. These assessment methods may include: (1) Cell
energy measurement that estimates the energy produced in cells, through measuring 12
changes in ATP and NADH levels. Changes in ATP and NADH levels can indicate changes
in OXPHOS pathway function; (2) Redox ratio can evaluate the balance between the
oxidation and reduction processes in cells. Imbalances may indicate abnormalities in
OXPHOS function; (3) Mitochondrial diameter measurement evaluates the size of
mitochondria in cells which indicates changes in the OXPHOS pathway function; and (4)
Mitochondrial protein distribution ratio measurement can test the distribution ratio of
mitochondrial proteins in cells, therefore can show the abnormalities in OXPHOS function.
The results from assessing cellular function and energy can help identify abnormalities
and dysregulation in the OXPHOS pathway. This assessment also allows researchers to
evaluate the impact of these changes on the OXPHOS system and to develop solutions to
improve cellular and body health. Through the evaluation of cellular functions and energy,
it provides information on cellular and energy metabolism that can reveal changes in the
OXPHOS pathway due to disease or genetic mutations and can be used to evaluate the
efficacy of potential therapeutic interventions on cellular and mitochondrial functions.
Moreover, this technique allows for the assessment of various components of
mitochondrial function, including oxygen consumption, ATP synthesis, and electron
transport chain activity. Enables the monitoring of changes in mitochondrial function over
time and under different experimental conditions is also a bonus point for this technique.
However, this can be technically challenging and require specialized equipment and
expertise. The sensitivity and specificity of these methods can be affected by various
factors, such as sample quality and the presence of contaminants. Next, measurements can
be affected by extracellular factors, such as oxygen and nutrient availability, which can
vary in different experimental conditions. 4. CONCLUSION
In conclusion, oxidative phosphorylation plays a key role in the whole cellular respiration
process, where combined action of the electron transport chain and chemiosmotic
coupling result in ATP production. It is regulated by a cell's energy needs, and thus the 13
ratio of ADP to ATP. Since the abnormality and dysregulation, there are some techniques
used to detect, which are by analyzing the functional domain of the OXPHOS pathway,
by evaluation of genes or proteins in the human body, or by estimating the function and
energy level of cellular. However, besides the benefits of each method, there are still
disadvantages in applying treatment. 14 REFERENCES
Alberts B, Johnson A, Lewis J, Raff M, Roberts K, Walter P (2002). The molecular biology
of the cell. Garland Science, New York.
Al-Dhabi, N. A., Ponmurugan, K., & Maran Jeganathan, P. (2017). Development and
validation of ultrasound-assisted solid-liquid extraction of phenolic compounds
from waste spent coffee grounds. Ultrasonics sonochemistry, 34, 206–213.
Biology Dictionary. (2017). Oxidative Phosphorylation. Biology Dictionary.
Demczko, M. (2023, April 18). Mitochondrial Oxidative Phosphorylation Disorders.
Finsterer, J. (2010). Treatment of central nervous system manifestations in mitochondrial disorders. European Journal of Neurology, 18(1), 28–38.
https://doi.org/10.1111/j.1468-1331.2010.03086.x
Klaus Hoffmeier. (2020). Cellular Respiration. Cellular Respiration | Microbiology.
Lehninger, A., L. Nelson, D., & M. Cox, M. (2008). Lehninger-Principles of Biochemistry.
Springer-Lehrbuch. https://doi.org/10.1007/978-3-662-08289-8 Libretexts. (2022). 8.3: Cellular Respiration. Biology LibreTexts.
https://bio.libretexts.org/Bookshelves/Microbiology/Microbiology_(OpenStax)/0
8%3A_Microbial_Metabolism/8.03%3A_Cellular_Respiration
Margarida Azevedo. (2015). Researchers Reduce Mutated Mitochondrial Genomes Implicated in Mitochondrial Diseases.
https://mitochondrialdiseasenews.com/2015/04/24/researchers-reduce-
mutatedmitochondrial-genomes-implicated-mitochondrial-diseases/
Merck Manuals Professional Edition.
Park, J.-S., Davis, R. L., & Sue, C. M. (2018). Mitochondrial dysfunction in Parkinson’s
disease: New mechanistic insights and therapeutic perspectives. Current
Neurology and Neuroscience Reports, 18(5). https://doi.org/10.1007/s11910-018- 0829-3 15
Reisch, A. S., & Elpeleg, O. (2007). Biochemical assays for mitochondrial activity: Assays
of TCA cycle enzymes and PDHC. Mitochondria, 2nd Edition, 199–222.
https://doi.org/10.1016/s0091-679x(06)80010-5
The Editors of Encyclopaedia Britannica. (1998, July 20). Cellular respiration | Definition,
Equation, Cycle, Process, Reactants, & Products. Encyclopedia Britannica.
https://www.britannica.com/science/cellular-respiration
Weinberg, F., & Chandel, N. S. (2009). Mitochondrial metabolism and cancer. Annals of the New York Academy of Sciences, 1177(1), 66–73.
https://doi.org/10.1111/j.1749-6632.2009.05039.x 16