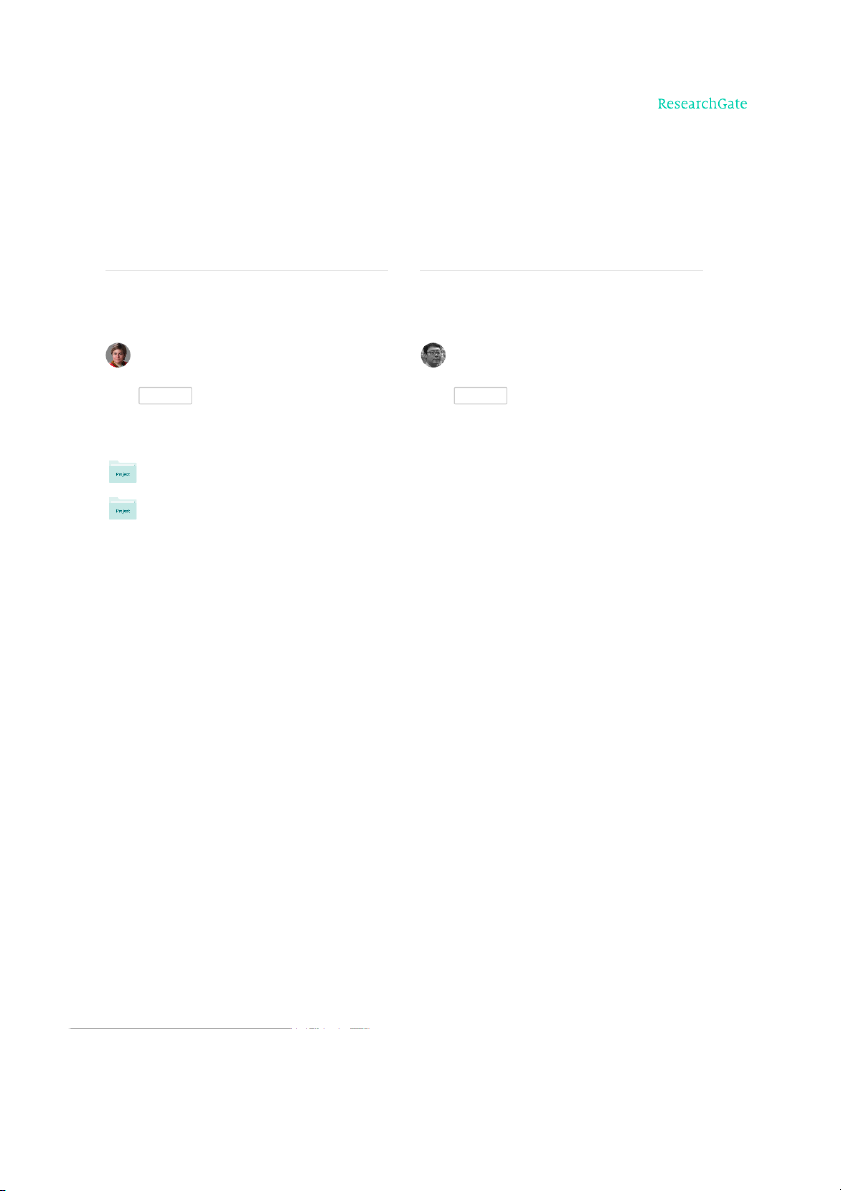
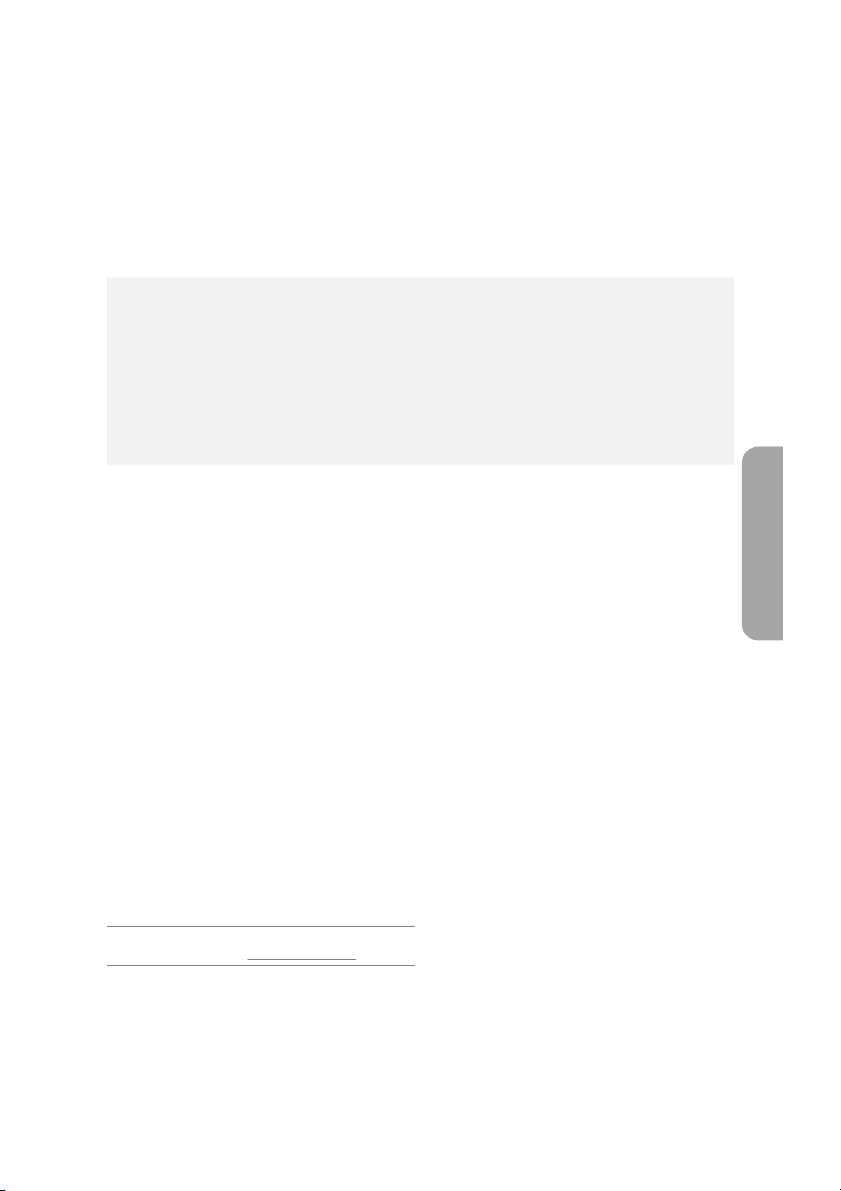
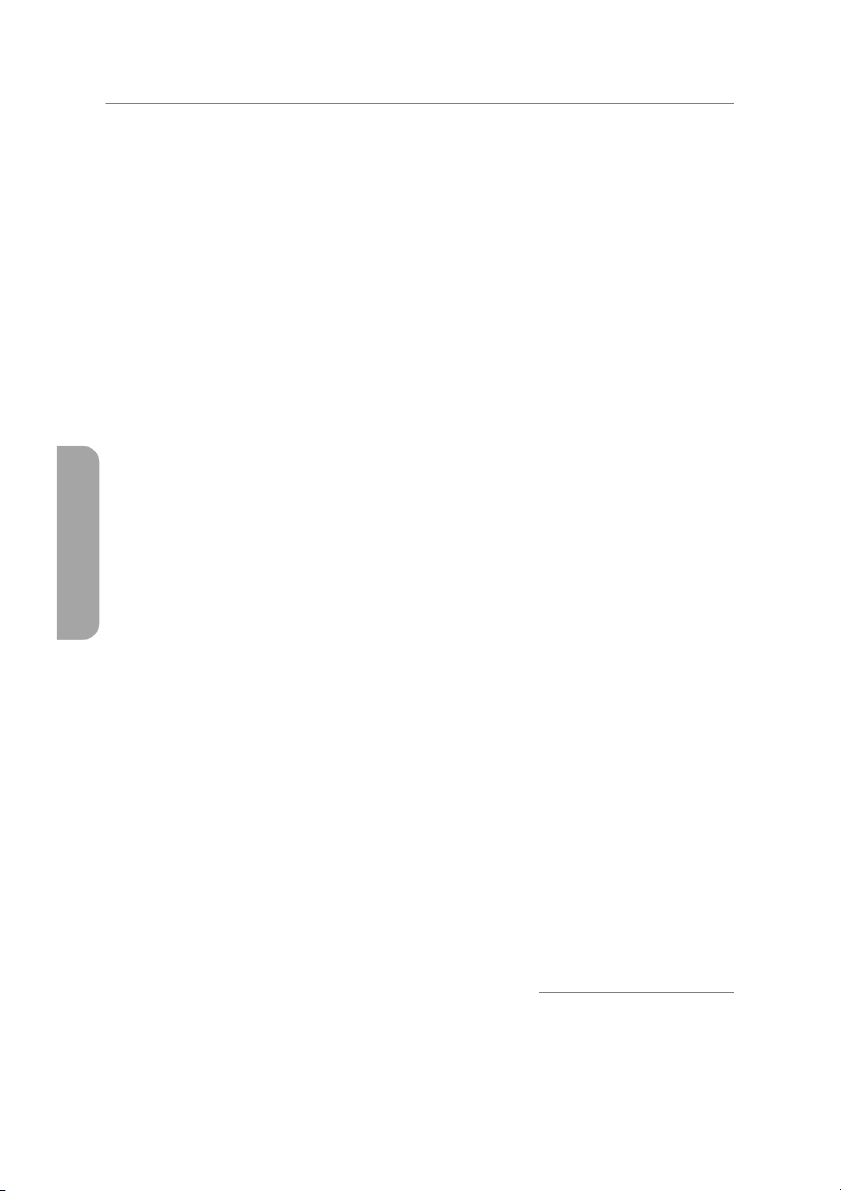
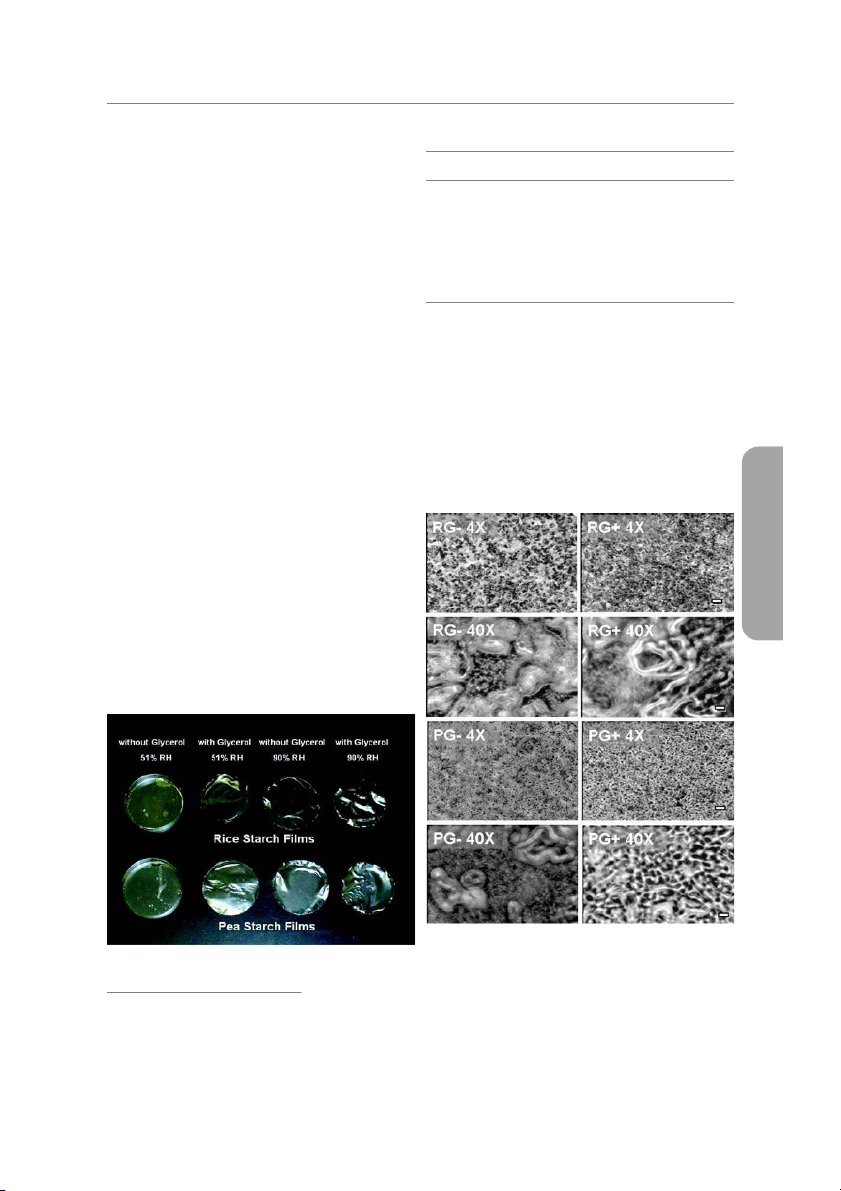
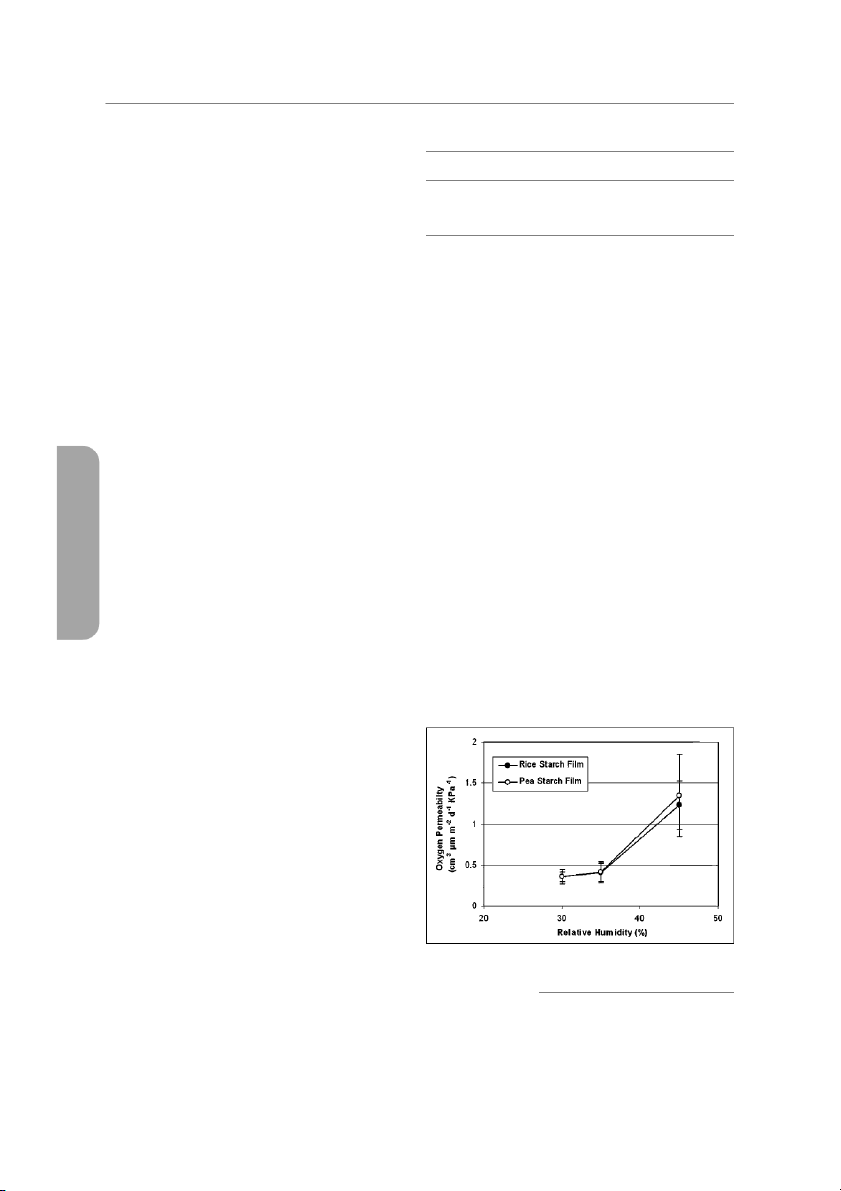
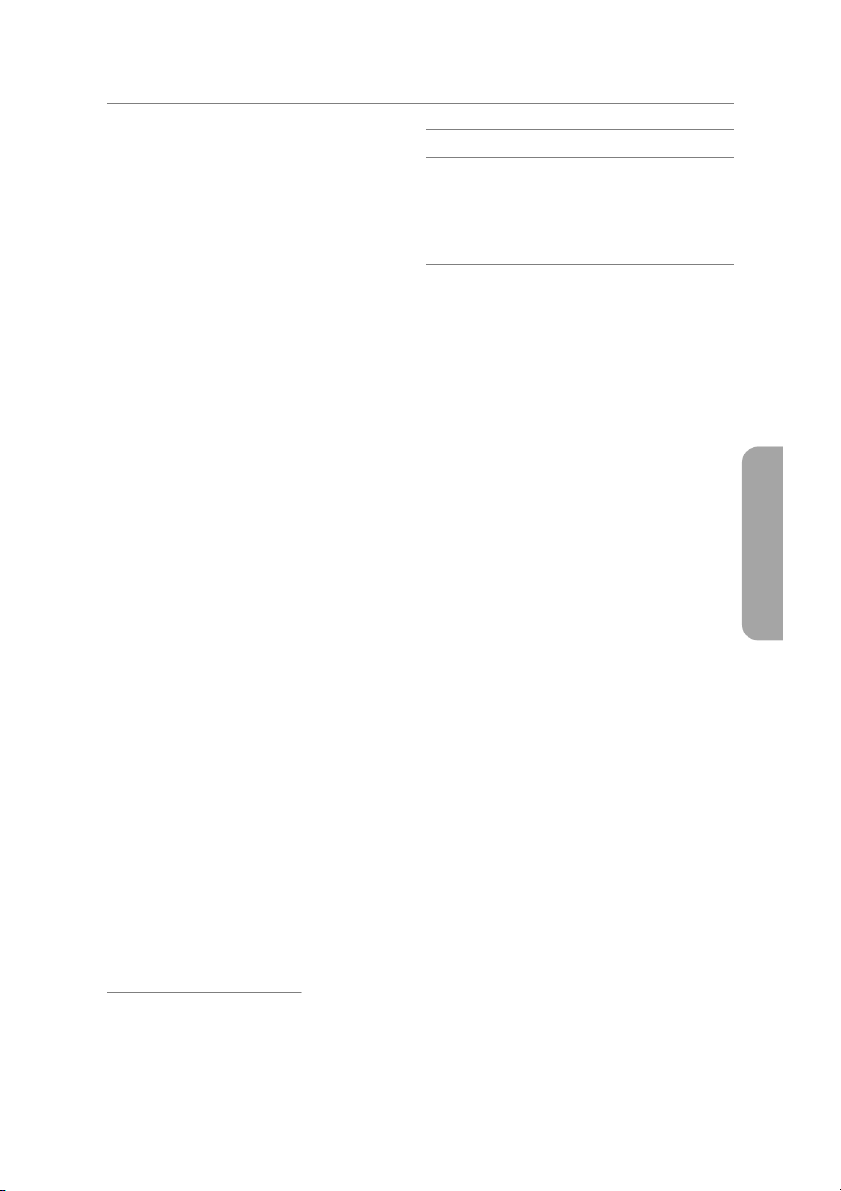
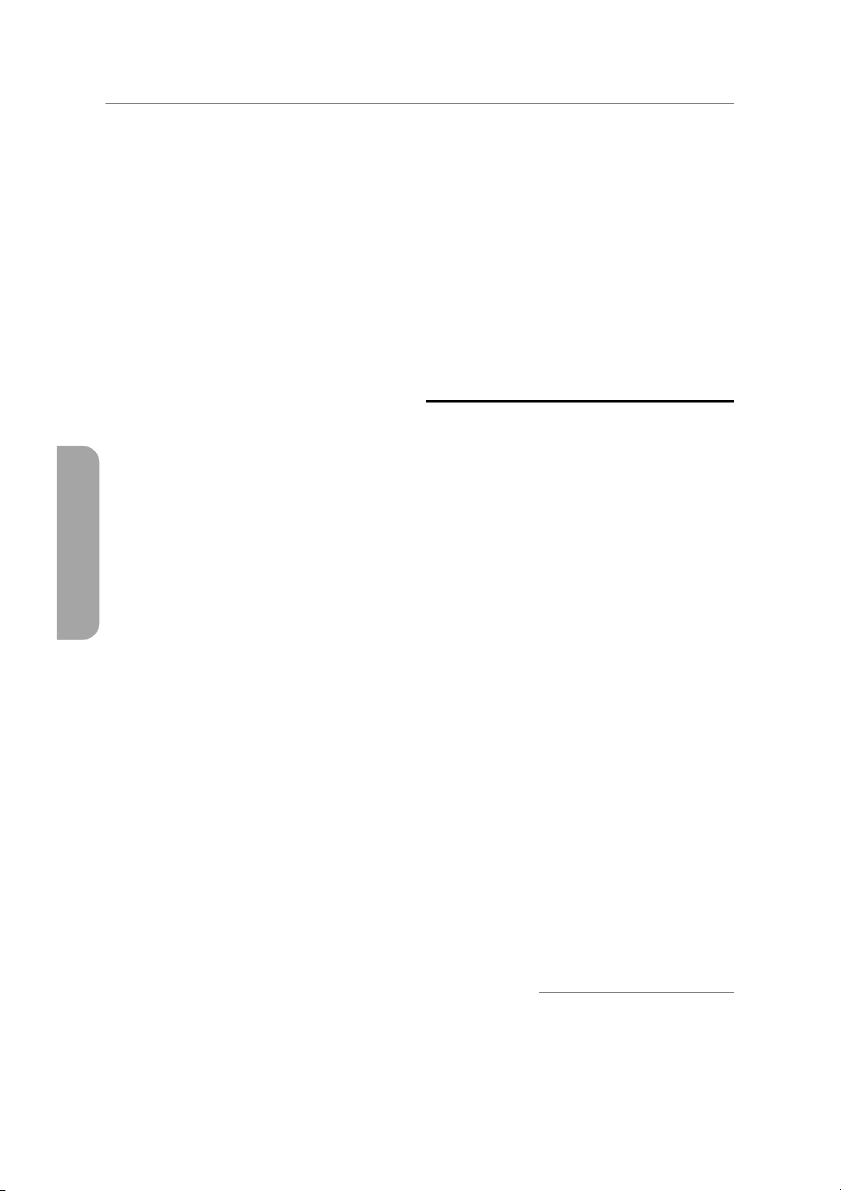
Preview text:
See discussions, stats, and author profiles for this publication at: https://www.researchgate.net/publication/284416178
Physical and mechanical properties of edible films made from high-amylose rice and pea starches Article · January 2003 CITATION READS 1 959 2 authors: Ghadeer F Mehyar Jung H. Han University of Jordan Pulmuone Food USA
39 PUBLICATIONS704 CITATIONS
59 PUBLICATIONS3,431 CITATIONS SEE PROFILE SEE PROFILE
Some of the authors of this publication are also working on these related projects:
Effectiveness of trisodium phosphate, lactic acid and commercial antimicrobials against pathogenic bacteria on chicken skin View project
Development of antimicrobial edible coatings to reduce microbial contamination of broiler carcasses View project
All content following this page was uploaded by Ghadeer F Mehyar on 27 December 2015.
The user has requested enhancement of the downloaded file.
JFS E: Food Engineering and Physical Properties
Physical and Mechanical Properties of High-
amylose Rice and Pea Starch Films as Affected
by Relative Humidity and Plasticizer G.F. MEHYAR AND J.H. HAN
ABSTRACT: The tensile properties, water vapor permeability, oxygen permeability at different relative humidities
(RH), and water solubility of edible films made of high-amylose rice starch (RS) or pea starch (PS) were mea-
sured and compared with the most commonly used edible films. Photomicrography of starch films shows amy-
lopectin-rich gels and amylose-rich granules. The addition of glycerol into starch films made amylose-rich
granules swollen and continuously dispersed between amylopectin-rich gels. Tensile strength of RS and PS
films decreased when RH increased from 51% to 90%, whereas elongation-at-break (E) of both films increased
when RH increased. Water vapor permeabilities of both films were similar, resulting in 130 to 150 g mm/m2/d /
kPa. Oxygen permeability of RS and PS were very low (< 0.5 cm3 m/m2/d/kPa) below 40% RH, and 1.2 to 1.4 at
45% RH. Water solubility of PS film was 32.0%, which is lower than that of RS film (44.4%). Overall high-amylose
rice and pea starch films possess an excellent oxygen barrier property with extremely high stretchability.
Keywords: starch film, edible film, oxygen permeability, water vapor permeability, tensile property Introduction
pea and rice flours. Most starches contain about 25% amylose and
The interest in biodegradable packaging such as edible films 75% amylopectin (Haase 1993; BeMiller and Whistler 1996). Starch is
and coatings is increasing because they consist of natural ma-
an attractive raw material for edible packaging because of its low
terials, which do not contribute to environmental pollution (Ahve-
cost, renewability, and biodegradability (Guilbert 2000). Further-
nainnen 1997; Webb-Jenkins 2002). These films and coatings may
more, it is abundant and nonallergic and possesses good mechanical
be applied on a food or between heterogeneous food components
and barrier properties that may allow for wider food applications
to prevent mass transfer phenomena that could deteriorate the
(Lloyd and Kirst 1962; Gujska and others 1994; Rindlav-Westling and
quality of foods (Guilbert 2000). Because edible films and coatings
others 1998; Pagella and others 2002). Films made of corn starches
are both a packaging and food component, they should have prop-
with high-amylose content showed excellent oxygen barrier proper-
E: Food Engineering & Physical Properties
erties that fulfill certain requirements of both packaging and foods.
ties, lower water solubility, lower retrogradation temperature, and
Edible packaging should be tasteless and free of toxic substances;
more stable mechanical properties at high RHs compared with those
serve as a barrier to water, gasses, and aroma; and have mechani-
made of normal starches (Lawton 1996; Rindlav-Westking 1998;
cal properties that allow efficient handling of the food products
Stading and others 2001). High-amylose starch is a very useful film-
(Krochta and De Mulder-Johnson 1997). It could be used as a car-
forming material because of its strong gelation properties and helical
rier or encapsulating material for functional ingredients such as
linear polymer structure (Juliano 1985; Elliasson and others 2001).
antioxidants, antimicrobial agents, pigments, or nutritional sub-
High-amylose corn starch that contains more than 50% amylose re-
stances contributing to microbial and chemical stability and nutri-
quires a very high temperature (> 130 °C) to be gelatinized (Case and
tional quality of the packaged foods (Krochta and De Mulder-
others 1998; Fredriksson and others 1998). Therefore, it is essential
Johnson 1997; Natrajan and Sheldon 2000).
to use a specially designed high-pressure vessel with continuous
Protein-based films and coatings such as those based on pea
agitation for the starch gelatinization. Compared with high-amylose
protein, soy protein, and whey protein have received increased
corn starch, high-amylose rice starch and pea starch contain 30%
interest in the recent years (Choi and Han 2001; Choi and Han
and 40% amylose, respectively, and accordingly a low gelatinization
2002; Krochta 2002). These films have good gas barrier properties,
temperature (< 90 °C). Therefore, simple water boiling units can pro-
mechanical properties, and nutritional qualities that allow them to
duce gelatinized starch dispersion.
either substitute the synthetic packaging materials or simplify the
Compared with the large amount of research and development
total packaging requirements (Gontard and others 1996; Mujica-
in the area of edible packaging, commercialization studies are still
Paz and Gontard 1997; Choi and Han 2001), whereas their applica-
few in number, even though the potential of a huge market exist
bility in foods was countered by their high cost and allergenicity
(Anonymous 2003). Starch-based films are sensitive to the sur- (Choi and Han 2002; Han 2002).
rounding relative humidity, which may affect their barrier and
Starch is the major constituent of legumes and cereals including
mechanical properties when they are used in food with high water
activity (Forssell and others 2002). The applicability of edible films
MS 20040116 Submitted 2/25/04, Revised 4/7/04, Accepted 7/22/04. The au-
and coatings to the foods depends on their barrier properties and
thors are with Dept. of Food Science, The Univ. of Manitoba, Canada. Direct
their ability to improve mechanical integrity of the foods. Because
inquiries to author Han (E-mail: hanjh@ms.umanitoba.ca).
high-amylose starch films have properties superior to those made
© 2004 Institute of Food Technologists
Vol. 69, Nr. 9, 2004—JOURNAL OF FOOD SCIENCE E449
Further reproduction without permission is prohibited Published on Web 10/28/2004
Physical and mechanical properties of starch films . . .
of normal starches, the objectives of this study were to determine
method D 82-91 (ASTM 1991). Initial grip distance and crosshead
the basic physical and mechanical properties of high-amylose rice
speed were 5 cm and 100 mm/min, respectively. TS was calculated
starch and pea starch films and to identify the effect of a plasticizer
by dividing the peak load by the cross-section area (thickness × 1
on the properties. The primary goal of our work was to identify the
cm) of the initial specimen. E was expressed as the percentage of
film-forming mechanisms of starch films during the dehydration
change in the length of the specimen to the original length between process.
the grips (5 cm). TS and E were obtained from 3 replications. After
RH conditioning, the moisture content of films were determined by Materials and Methods
measuring the weight difference of film samples before and after
dehydration at 105 °C for 24 h. Materials
High-amylose rice starch (RS) and pea starch (PS) was supplied
Water vapor permeability
by A & B ingredients (Fairfield, N.J., U.S.A.) and Parrheim Foods Co.
Choi and Han (2001) procedure tailored from modified ASTM
(Portage-la-Prairie, Man., Canada), respectively. The rice starch and
E96-92 gravimetric method of McHugh and others (1993) was used
pea starch contained approximately 30% and 40% amylose, respec-
to determine the water vapor permeability (WVP) and RH underside
tively. Glycerol from Sigma Chemical Co. (St. Louis, Mo., U.S.A.)
the film. Distilled water (10 mL) was dispensed into flat-bottom was used as the plasticizer.
acrylic (Plexiglas®) cups with wide rims. The cups were covered with
the films, sealed with a ring and silicon sealant (High Vacuum Grease, Film preparation
Dow Corning, Midland, Mich., U.S.A.), and placed in a desiccator
Aqueous dispersion of 3% (w/w) RS or PS were prepared, and
cabinet equipped with fans and a digital hygrometer to be held a
glycerol was added at a ratio of 1:2 (w/w) of glycerol to starch. For
constant humidity at 25 °C. Weight changes were measured period-
the reference tests, pure rice and pea starch films were produced
ically after the steady state of the weight loss was achieved and used
without containing glycerol. The solutions were heated with con-
to calculate the resulting water vapor transmission rate (WVTR) and
tinuous mixing until boiling and kept at the boiling temperature
the %RH underneath the testing film. Relative humidity underneath
for 15 min to allow complete gelatinization of the starch granules.
the film was calculated from free diffusion of moisture in air inside the E
The film-forming solutions were cooled to approximately 60 °C and
cup and the distance from water surface to test film following the : Fo
then cast into polystyrene petri dishes (10-cm dia) by pouring 12 g
procedure of McHugh and others (1993) using visual basic program od
per dish resting on a leveled smooth surface. The petri dishes were
developed by J.H. Han The WVP was calculated as follows: Eng
kept at room temperature for 24 h to allow the film-forming solution ine
to dry before the next stage of experiments. The thickness of the WVP = WVTR × Thickness/⌬P e H2O rin
films was measured with a caliper micrometer (B.C. Ames Co., Walth- g &
am, Mass., U.S.A.) at 5 random positions of the film after the films
The ⌬PH2O is the partial pressure difference of water vapor between Ph
were peeled off. The average thickness was used for the proceeding the 2 sides of the film. ysi
mechanical test, water vapor permeability, and oxygen permeabil- cal
ity determination. The films were transferred into large plastic bags Oxygen permeability Pr
(lubricant-free low density polyethylene [LDPE] sandwich bags)
The oxygen transmission rate (OTR) was measured using OX-TRAN ope
and arranged side by side into 1-layer thickness. The filled bags
2/61 (Mocon, Minneapolis, Minn., U.S.A.). The measurements were rtie
were then gently pressed on both sides of the films, which allowed
done at 23 °C and the films were equilibrated into RH of 30%, 35%, s
both sides to stick onto the bag to minimize the access of air or mois-
and 45% during the experiment. The oxygen permeability (OP) was
ture to the films. The bags were then sealed and stored at room
calculated by the following equation:
temperature until the day of testing. The storage period of the films was less than 1 wk. OP = OTR × Thickness / ⌬PO2 Photomicrography
The ⌬PO2 is the partial pressure difference of oxygen between the 2
After drying for 24 h, the films on the petri dishes were condi-
sides of the film, which was 1 atm during the experiment.
tioned for 5 d in 51% and 90% RH over the supersaturated salt so- lutions of Ca (NO
Percentage of soluble matter
3) 4H2O and Zn SO4 7H2O, respectively, at room
temperature. The petri dishes containing the films were placed on
Film specimens (1.5 × 1.5 cm) were dried for 3 d in a desiccator over
an inverted phase-contrast microscope (PCM, Nikon Diaphot,
anhydrous calcium sulfate. The specimens were accurately weighed to
Kanagawa, Japan) equipped with a TV camera (Panasonic WV-
obtain the initial dry weight (Wi). The dried specimens were immersed
1550), and the surface structure of the films were observed. With 4×
in test tubes containing 5 mL distilled water. The tubes were covered
and 40× magnitude lenses, the image of film surface was recorded
with aluminum foil and incubated at ambient temperature for 24 h
by a personal computer equipped with a video capture card. The
with occasional gentle agitation. The films then were dried in the oven
image resolution was set at 640 × 480 pixels.
at 70 °C for 24 h and weighed to obtain the final dry weight (Wf). The
percentage of soluble matter (% SM) of the films was calculated using Tensile test the following equation:
The films stored in plastic bags were cut in 1- × 8-cm strips and
conditioned in a desiccator for 3 d at 51% or 90% RH. In the case of
% SM of the films = [(Wi – Wf)/Wi] × 100
the nonplasticized starch films, the pure rice and pea starch films
were humidity-conditioned before being peeled off the petri dish-
es. Tensile strength (TS) and elongation-at-break (E) were deter- Results and Discussion
mined from a stress-strain curve using a texture analyzing instru-
ment ( Texture Analyzer, TA-XT2, Texture Technologies Corp., Film preparation
Scarsdale, N.Y., U.S.A.) following the procedure outlined in ASTM
After drying the film-forming solutions, the glycerol-plasticized E450
JOURNAL OF FOOD SCIENCE—Vol. 69, Nr. 9, 2004
URLs and E-mail addresses are active links at www.ift.org
Physical and mechanical properties of starch films . . .
films were easily peeled off from the petri dishes, whereas RS films
Table 1—Moisture content (MC), tensile strength (TS), and
were much stickier than PS films. Both films were translucent,
elongation-at-break (E) of starch films at 51% and 90% relative humidity
strong, and flexible enough to be easily handled. Nonplasticized
films containing pure rice and pea starch were too brittle to be RH M C TS E Thickness
peeled off from the petri dishes. However, the films conditioned in Film (%) (%) (MPa) (%) ( mm )
a 90% RH chamber were flexible and easy to peel off. Figure 1 A. With glycerol
shows rice and pea starch films with and without glycerol at 51% Rice starch 51 27 3.2 ± 0.30 265.6 ± 13.60 0.071 90 43 1.9 ± 0.24 751.4 ± 20.60 0.077 and 90% humidity. Pea starch 51 27 4.2 ± 0.44 323.7 ± 12.00 0.072 90 44 2.8 ± 0.24 420.9 ± 93.23 0.075 Photomicrography B. Without glycerol
At 4× magnitude, regardless the incorporation of glycerol, RS and Rice starch 51 11.4 — — 0.045
PS films possess 2 phases: One as a starch gel matrix and the other 90 27 12.1 ± 0.23 332 ± 33.5 0.069
as black porous structure. RS film has bigger gel matrix particles and Pea starch 51 11.6 — — 0.052
pores than those of PS films. Particularly, RS film containing glycerol 90 28 3.0 ± 0.31 150 ± 8.3 0.073
has smaller gel matrix particles than nonplasticized films. These
large gel matrix particles were dried from swollen particles of starch
granules. Amylopectin, a major polymer of starch, forms gel parti-
cles during starch gelatinization while amylose was excluded from
starch granules (Goodfellow and Wilson 1990). Therefore, these Moisture content
large gel particles that were assumed to be swollen starch granules
Plasticized films contain more moisture than nonplasticized
in 4× magnitude images are assumed to be an amylopectin-rich
films, and the films conditioned at 90% RH possessed higher MC
phase. At 40× magnitude, it was observed that the black pores of
than films in 51% RH (Table 1). The moisture content (MC) of plas-
nonplasticized RS and PS films were filled with very small gel gran-
ticized RS and PS films conditioned at 51% RH (that is, 27% MC)
ules of which size were estimated as < 2-m dia. Amylose is exclud-
had the same MC of nonplasticized RS and PS films at 90% RH,
ed from starch granules during gelatinization of amylopectin and
while they contained 11.4% to 11.6% MC at 51% RH. The plasticizer
form small granular aggregations (Zobel 1984). These small gran-
(glycerol) in RS and PS films held 15.4% to 15.6% more water than
ules in 40× magnitude images were assumed to be amylose-rich
aggregations. When RS films have been plasticized by glycerol,
these small granules in amylose-rich phase (RG-40× in Figure 2)
were swollen and formed opaque matrix (RG+40× in Figure 2).
Therefore, the structure of plasticized films became more connec-
tive in the amylose-rich phase. In PS films, the big gel matrix (amy-
lopectin-rich phase) and the small granules (amylose-rich aggrega-
tions) in PG-40× (Figure 2) disappeared and formed more
homogeneous film structure in PG+40×, when the PS films were
plasticized (Figure 2). It was concluded that the addition of plasti-
cizers into starch films interferes with the aggregation of amylopec-
tin and amylose as well as phase separation of both polymers, and
E: Food Engineering & Physical Properties
forms more homogeneous structure of amylose and amylopectine mixture.
Figure 2—Photomicrography (4× and 40× magnitude) of rice
(R) starch and pea (P) starch films with (G+) and without
Figure 1—Rice and pea starch films after relative humid-
(G–) glycerol at 51% relative humidity (RH). Scale bars in ity conditioning
4× and 40× are 100 m and 10 m, respectively.
URLs and E-mail addresses are active links at www.ift.org
Vol. 69, Nr. 9, 2004—JOURNAL OF FOOD SCIENCE E451
Physical and mechanical properties of starch films . . .
nonplasticized films after conditioning at 51% RH. Glycerol is a
Table 2—Water vapor permeability (WVP) and water solu-
good water-holding agent and increased the amount of water in RS
bility (soluble matters, SM) of starch films containing glyc- erol
and PS films as well as serving as a plasticizer. Film WVP (RH)* SM (%) Tensile test (g mm/m2/d/kPa) with glycerol
The tensile strength (TS) and elongation at the break (E) of plas- Rice starch 130.93 ± 21.08 44.4 ± 4.39
ticized high-amylose RS and PS films at 2 RHs are shown in Table 1, (30.0%/72.9%)a (1.48 ± 1.001)b
part A. At the same RH, plasticized PS films had higher TS than plas- Pea starch 150.31 ± 44.93 32.0 ± 3.35
ticized RS films. The TS of both films decreased by increasing the (30.0%/72.0%)a (1.50 ± 0.832)b
RH from 51% to 90%, whereas E of both films increased with in-
aRelative humidity (RH) of outside/inside cups (top/underneath of films containing glycerol).
creasing RH. The E of PS at 90% had very large standard deviation
bReference SM of nonplasticized pure starch film without glycerol.
compared with other films. When RS and PS were not plasticized by
glycerol, free-standing alone RS an PS films were not produced at
51% RH. At 90% RH, nonplasticized RS films were stronger and more
extendable (in Ts and E, respectively) than nonplasticized PS films
higher concentration of amylose in PS films may allow a higher sta-
(Table 1, part B). Comparing TS and E values of 90% RH condi-
bility and subsequently less sensitivity in E by increasing RH, than
tioned nonplasticized pure starch films to glycerol-plasticized films, RS films (Table 1, part A).
the addition of plasticizer decreased TS of RS films and E of both RS
and PS films. TS of PS films was not affected by plasticizers at 90%
Water vapor permeability
RH. Moisture obtained by PS films from 90% RH may contribute to
The WVPs of plasticized RS and PS films were high and ranged be-
the plasticization effectively.
tween 130 to 150 g mm/m2/d /kPa (Table 2). With 21 to 45 g mm/m2/d/
Rindlav-Westling and others (1998) found that stress at break de-
kPa of standard deviation, there was not a big difference in WVP be-
creases and strain at break increases by increasing RH from 20% to 70%
tween RS and PS (131 compared with 150 g mm/m2/d/kPa). The hydro-
for amylose films plasticized with glycerol (2.5:1 of polymer to glycerol).
philic nature of both starch molecules made the film easily attracted by E
The elastic modules of both amylose and amylopectin films decreased
water. The water molecules can easily diffuse through the amylopectin : Fo
by increasing RH from 20% to 80% (Stading and others 2001). In corn
films than the amylose films (Rindlav-Westling 1998). Our RS and PS od
films contain the higher proportion of amylopectin, approximately
starch, increasing the RH from 35% to 65% resulted in a reduction in Eng
strength of about 43% (Lloyd and Kirst 1962). Increasing the plasticizer
70% and 60%, respectively, which make it a poor barrier for water. Al- ine
levels in edible films was generally found to increase E and decrease TS
though there is a 10% difference in amylose/amylopectin content be- eri
tween RS and PS, the WVP of both films did not show any difference. n
(Choi and Han 2001). As with other starch films (Lloyd and Kirst 1962; g &
Rindlav-Westling and others 1998; Stading and others 2001) and pro-
The WVP of PS showed nearly twice of the coefficient of variance Ph
tein films (Choi and Han 2001), RS and PS films conditioned at high
(C.V. = 29.9%) to the C.V. of RS (16.1%). It was found that inclusion of ys
RH (90%) were weaker but more flexible than those conditioned at low
hydrophobic materials such as edible fatty acids, protein-based mate- ical
RH (51%) as shown in Table 1, part A. Obviously, high RH led to high
rials, or waxes in hydrophilic edible films can retard water diffusivity Pr
moisture content, of which water molecules presumably act as a typ-
through the film and improve its water barrier properties (Krochta and ope
ical plasticizer for the starch films. In addition to the typical plasticiza-
De Mulder-Johnston 1997; Ayranci and Tunc 2001). Chemical mod- rtie
tion effect, too many water molecules in the starch films could cause
ification of the polymer structure such as increasing the crosslinkings s
a looser and heterogeneous structure that weakens the film
showed promising in improving film water barrier properties (Sebti and
strength (Stading and others 2001). Regardless of RH, MC, and film others 2002).
thickness, plasticized PS films showed overall stronger mechanical
properties than plasticized RS films (Table 1), mainly because of Oxygen permeability
their higher amylose content (Lloyd and Kirst 1962). In addition,
Plasticized RS and PS films were excellent oxygen barriers
the change in TS and E of PS films due to the changes in RH is less
significant than those of RS films, which again evidences the envi-
ronmental stability of high-amylose starch films in terms of me-
chanical properties (Lawton 1996).
The RS films contained lower concentrations of amylose than PS
films. Amylose is the helical linear polymer of the films and responsible
for keeping the elongation properties of high-amylose starch films.
Lloyd and Kirst (1962) found that TS of corn starch films increased
with increasing its content of amylose at the same RHs. The high-
amylose films were the most resistance to the change in E by increas-
ing RHs from 20% to 90% when compared with films contained nor-
mal starches (Lawton 1996). Lourdin and others (1995) found that the
pure amylopectin films showed elongation behavior similar to that of
ductile material, whereas that of pure amylose films was similar to the
brittle material. They related this to possible difference in glass transi-
tion temperature (Tg) values at room temperature. Amylopectin films
have Tg value slightly lower than the room temperature while it is
much higher than the room temperature for amylose films. Amylose-
based films have more stable microstructure at higher RHs (> 50%)
with strong molecular orientation than those of amylopectin films
Figure 3—Oxygen permeability of rice starch and pea starch
(Rindlav-Westling and others 1998; Stading and others 2001). The
films containing glycerol at different relative humidity E452
JOURNAL OF FOOD SCIENCE—Vol. 69, Nr. 9, 2004
URLs and E-mail addresses are active links at www.ift.org
Physical and mechanical properties of starch films . . .
(OP < 0.5 cm3 m/m2/d/kPa) at RH below 35% (Figure 3). Increas-
Table 3—Comparison of the properties of edible films
ing the RH above 40% increased the oxygen permeability of both TSb SM
films to the range of 1.2 to 1.4 cm3 m/m2/d/kPa, which were still su- Filma (MPa) E (%) WVPc OPb,c (%)
perior oxygen barriers. At intermediate humidity above 40% RH, RS Rice starch 3.2 265.6 130.9 2.0 to 2.2 44.4
and PS films also showed larger standard deviation than those at Pea starch 4.2 323.7 150.3 1.5 to 1.8 32.0
low RH (Figure 3). Although OPs of RS film and PS film were not sig- Methylcellulosed 20 to 25 75 to 80 270 to 450 7.2 to 10.5 82.8
nificantly different (P < 0.01) in our experimental ranges of RHs, Hydroxypropyl
8 to 10 101 to 110 250 to 320 4.8 to 5.7 40 methylcellulosed
the PS films kept more barrier properties at high RH (45%). Forssell Whey proteine 6.9 199.0 119.0 1.0 30.0
and others (2002) found that amylose films plasticized with glycerol Soy proteine 5.0 86.0 91.2 6.1 35.1
have good oxygen barrier at RHs below 50% and glycerol content Pea proteine 7.3 31.9 98.0 — 38.7
up to 30%. Increasing the surrounding RH from 50% to 90% result- Wheat glutene 2.0 170.0 108.0 6.7 —
ed in increased oxygen permeability of both amylose and amy-
aEdible materials contain glycerol as a plasticizer at the ratio 2:1 of
lopectin films. However, amylose films kept more barrier properties biopolymer to glycerol.
bConditions for tensile strength (TS) and oxygen permeability (OP) are
than amylopectin films. Similar findings were obtained by Rindlav-
approximately 25 °C, 50% relative humidity (RH). OP data for rice and pea
starch films were estimated by extrapolation from Figure 3.
Westling and others (1998) when they found that amylose films
cUnits are g mm/m2/d/kPa for water vapor permeability (WVP) and cm3 m/
had lower OP than amylopectin films at RH between 35% and 75%. m2/d/kPa for OP.
dData from Park and others (1993) for TS, elongation-at-break (E), WVP, and
The network structure of amylose films is very stable at RH up to
OP, and Song and Kim (1999) for soluble matter (SM). WVP was determined at
50%. Increasing the RH above 50% resulted in increased mobility of
very low RH (0%/11%), whereas WVP values of other starch and protein films
were determined at higher RH (approximately 10%/70%).
oxygen molecules in the polymeric network structure and its perme-
eData collected from Krochta (2002) and Choi and Han (2001).
ability through the looser structure (Gontard and others 1996;
Rindlav-Westling and others 1998). The higher concentration of
amylose in RS and PS films may be responsible for oxygen barrier
properties, especially above intermediate RH.
and physical integrity. RS films showed similar properties at low Total soluble matter
RHs but they were weaker and more stretchable than PS film. Both
Plasticized RS and PS films had moderate solubility of 44% and
films loosen their oxygen barrier properties slightly with increasing
32%, respectively (Table 2). This may be caused by the incorporated
RH. The addition of glycerol increased solubility and moisture con-
soluble component, which is glycerol. The glycerol-to-starch ratio in
tent of starch films. The content of amylose in starch was used to
these films was 1:2. Therefore, the total loss of glycerol from the films
explain the strong film-forming properties of starch-based edible
may result in 33% of solubility. Compared with PS film, which had
films at different RHs. With the exception of WVP, RS and PS films
32% of solubility that was very close to glycerol content, RS film had
were either comparable or superior to other edible films such as
higher solubility (44%). This may reflect the fact that the starch net-
whey protein, soy protein, pea protein, and wheat gluten films.
work of RS film is partially soluble. From these solubility results, it was
Substitution of protein-based packaging materials with high-amy-
concluded that a pea starch network has higher interacting force with
lose starches in food applications is suggested. Development of
glycerol molecules as well as higher starch-starch intermolecular force
composite packaging materials containing high-amylose rice or pea
than a rice starch structure. If it were considered that PS possessed
starches with other biopolymers is also suggested to complete an
10% more amylose than RS, the increase in the intermolecular force
understanding of functional properties of the other constituents.
of PS would be caused by the interaction between amylose molecules
E: Food Engineering & Physical Properties
This may allow wider applications of films and coatings made of
of PS, which is hypothesized as a major film-forming mechanism of
high-amylose rice and pea starches.
starch films. For the nonplasticized pure starch films, SM ranged from
1.48% to 1.50%, and there is no difference in SM values between RS Acknowledgments
and PS films. Because nonplasticized starch films do not exhibit any
The authors thank Dr. Zhiqiang Liu in the Dept. of Food Science at
difference in SM whereas plasticized films have a difference in SM,
the Univ. of Manitoba for his contribution of discussing the exper-
it is concluded that the glycerol contributes toward an increase in SM imental data. of RS films.
Table 3 compared the physical and mechanical properties of RS References
and PS films to other carbohydrate and protein films at approxi-
Ahvenainen R, Myllarinen P, Poutanen K. 1997. Prospects of using biodegrad-
mately 25 °C, 50% RH. Compared with other modified cellulose
able protective films for foods. Eur Food Drink Rev 1997(Summer):73,75,77–
edible films, RS and PS films possessed 1.9 to 7.8 times higher TS, 80.
Anonymous. 2003. Environmentally-friendly packaging: a green cycle. Asia Pa-
2.4 to 4.3 times higher E, 2.2 to 7 times lower OP, and 1.7 to 3.5 times
cific Food Industr 15(1):24–25, 28.
lower WVP. The RS and PS films have 2 to 16 times higher E, 2 to 7
[ASTM] American Society for Testing Methods. 1991. Standard test method for ten-
sile properties of thin plastic sheeting. D882. In: Annual book of American So-
times lower OP, and 1.1 to 1.6 times higher WVP than edible protein
ciety for Testing Methods. Philadelphia, Pa.: ASTM. p 313–21.
films, whereas their solubility and TS were comparable to other
Ayranci E, Tunc S. 2001. The effect of fatty acid content on water vapour and car-
bon dioxide transmissions of cellulose-based films. Food Chem 72(2):231–6.
protein films. These properties potentiate applications in which
BeMiller JN, Whistler RL. 1996. Carbohydrates. In: Fennema OR, editor. Food chem-
higher elongation, intermediate water vapor permeability, and
istry. 3rd ed. New York: Marcel Dekker. p 157–223.
Case SE, Capitani T, Whaley JK, Shi YC, Trzasko P, Jeffcoal R, Goldfarb HB. 1998.
excellent oxygen barrier are required, such as with oxidation-sen-
Physical properties and gelation behavior of a low-amylopectin maize starch
sitive intermediate or dried foods.
and other high-amylose maize starches. J Cereal Chem 27(3):301–14.
Choi WS, Han JH. 2001. Physical and mechanical properties of pea-protein-based
edible films. J Food Sci 66(2):319–22. Conclusions
Choi WS, Han JH. 2002. Film-forming mechanism and heat denaturation effects
The low cost, ease of preparation, nonallergenicity, and superior on the physical and chemical properties of pea-protein-isolate edible films. J Food Sci 67(4):1399–406.
mechanical and barrier properties of the high-amylose PS and
Elliasson A, Tatham A. 2001. Cereal starches and proteins. In: Dendy DAV, Do-
RS films potentiate their applications for food preservation. PS
braszczyk BJ, editors. Cereals and cereals products: chemistry and technology.
Gaithesburg, Md.: Aspen Publisher.p 68–89.
films were strong and elastic and possessed a good barrier property
Forssell P, Lahtinen R, Lahelin M, Myllarinen P. 2002. Oxygen permeability of
URLs and E-mail addresses are active links at www.ift.org
Vol. 69, Nr. 9, 2004—JOURNAL OF FOOD SCIENCE E453
Physical and mechanical properties of starch films . . .
amylose and amylopectin films. Carbohydr Polym 47(2):125–9.
films and foams. Carbohydr Polym 27(4):261–70.
Fredriksson H, Silverio J, Andersson R, Eliasson AC, Aman P. 1998. The influence
McHugh TH, Avena-Bustillos R, Krochta JM. 1993. Hydrophilic edible films mod-
of amylose and amylopectin characteristics on gelatinization and retrograda-
ified procedure for water vapor permeability and explanations of thickness
tion properties of different starches. Carbohydr Polym 35(3):119–34.
effects. J Food Sci 58(5):899–903.
Gontard N, Thibault R, Cuq B, Guilbert S. 1996. Influence of relative humidity
Mujica-Paz H, Gontard N. 1997. Oxygen and carbon dioxide permeability of wheat
and film composition on oxygen and carbon dioxide permeabilities of edible
gluten film: effect of relative humidity and temperature. J Agric Food Chem
films. J Agric Food Chem 44(4):1064–9. 45(10):4101–5.
Goodfellow BJ, Wilson RH. 1990. A fourier transform IR study of the gelation of
Natrajan N, Sheldon BW. 2000. Inhibition of Salmonella on poultry skin using
amylose and amylopectin. Biopolymers 30:1183–9.
protein and polysaccharide-based films containing a nisin formulation. J Food
Guilbert S. 2000. Edible films and coatings and biodegradable packaging. Packag- Prot 63(9):1268–72.
ing of milk products. Bull IDF 346:10–6.
Pagella C, Spigno G, Faveri DM. 2002. Characterization of starch based edible
Gujska E, Duskiewicz-Reinhard W, Khan K. 1994. Physiochemical properties of
coatings. Trans I Chem E 80(9):193–8.
field pea, pinto and navy bean starches. J Food Sci 59(3):634–6.
Park HJ, Weller CL, Vergano PJ, Testin RF. 1993. Permeability and mechanical prop-
Haase NU. 1993. A rapid test procedure for estimating the amylose content of
erties of cellulose-based edible films. J Food Sci 58(6):1361–4.
pea starch. Plant Breeding 111:325–9.
Rindlav-Westling A. Stading M, Hermansson A, Gatenholm P. 1998. Structure,
Han JH. 2002. Protein-based edible films and coatings carrying antimicrobial
mechanical and barrier properties of amylose and amylopectin films. Carbohy-
agents. In: Gennadios A, editor. Protein-based films and coatings. Boca Raton, dr Polym 36(2):217–24. Fla.: CRC Press. p 485–99.
Sebti I, Ham-Pichavant F, Coma V. 2002. Edible bioactive fatty acid-cellulosic de-
Juliano BO. 1985. Criteria and tests for rice grain qualities. In: Juliano BO, editor.
rivative composites used in food packaging applications. J Agric Food Chem
Rice: chemistry and technology. St. Paul, Minn.: The American Assn. of Cereal 50(15):4290–4. Chemistry. p 443–524.
Song TH, Kim CJ. 1999. Effect of calcium addition on physicochemical properties
Krochta JM. 2002. Proteins as raw materials for films and coatings: definitions,
of cellulose-based edible films. Kor J Food Sci Technol 31(1):99–105.
current status, and opportunities. In: Gennadios A, editor. Protein-based films
Stading M, Rindlav-Westling A, Gatenholm P. 2001. Humidity-induced structur-
and coatings. Boca Raton, Fla.: CRC Press. p 1–41.
al transitions in amylose and amylopectin films. Carbohydr Polym 45(3):209–
Krochta JM, De Mulder-Johnston C. 1997. Edible and biodegradable polymer films: 17.
challenges and opportunities. Food Technol 51(2):61–74.
Webb-Jenkins J. 2002. Biodegradable packaging for the green consumer. Fruit Proc
Lawton JW. 1996. Effect of starch type on the properties of starch containing films. 12(6):268–9. Carbohydr Polym 29(3):203–8.
Zobel HF. 1984. Gelatinization of starch and mechanical properties of starch
Lloyd NE, Kirst LC. 1962. Some factors affecting the tensile strength of starch films.
pastes. In: Whistler RL, BeMiller JN, Paschall EF, editors. Starch: chemistry and Cereal Chem 40(3):154–61.
technology. 2nd ed. New York: Academic Press. p 285–309.
Lourdin D, Della Valle G, Colonna P. 1995. Influence of amylose content on starch
E: Food Engineering & Physical Properties E454
JOURNAL OF FOOD SCIENCE—Vol. 69, Nr. 9, 2004
URLs and E-mail addresses are active links at www.ift.org View publication stats